Contents
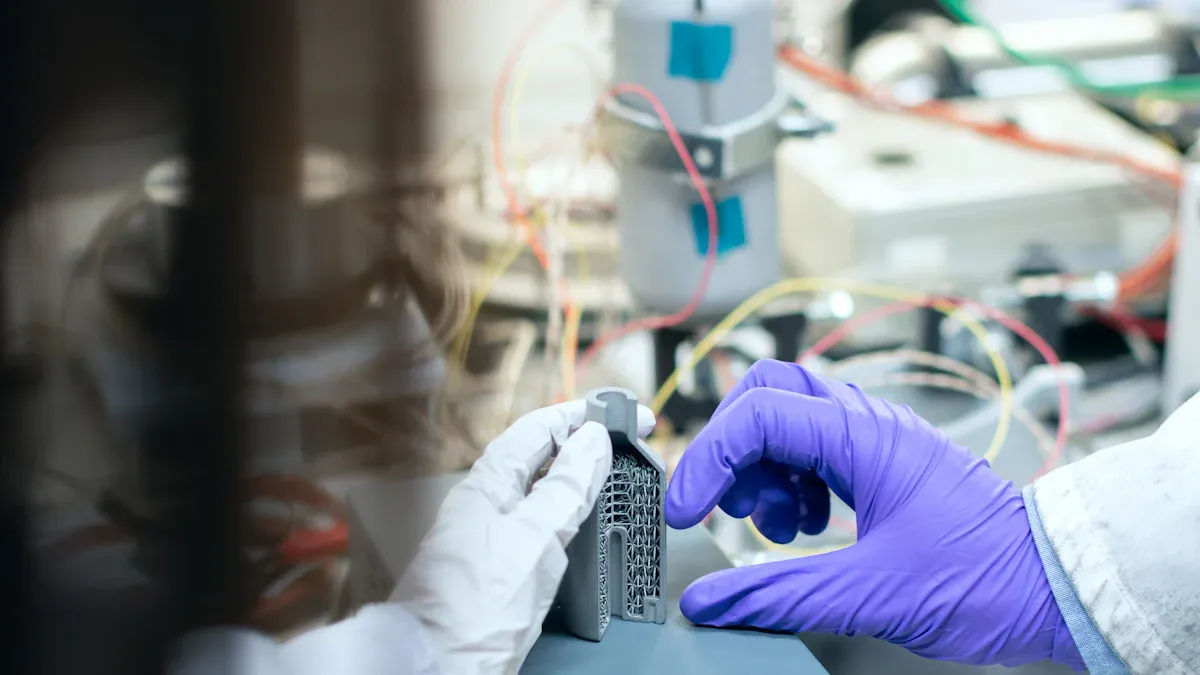
Lithium-ion batteries are crafted through a meticulous process that combines specific materials such as lithium, cobalt, nickel, manganese, and graphite. Each of these materials plays a critical role in optimizing the battery’s performance and lifespan. The key components—cathode, anode, electrolyte, and separator—are precisely engineered using these materials, showcasing the intricate process of how lithium-ion batteries are made. This understanding highlights why these batteries have become the leading choice for modern energy storage, powering a wide range of devices from smartphones to electric vehicles.
Key Takeaways
Lithium-ion batteries are made with materials like lithium, cobalt, and graphite. These materials help the battery work well and last longer.
The cathode material changes how much energy the battery holds and its voltage. Materials like lithium cobalt oxide and lithium iron phosphate have different advantages.
Anode materials, such as graphite and silicon, affect how fast the battery charges and how much energy it stores. Silicon can make the battery work better.
Electrolytes help lithium ions move and can be liquid or solid. Solid electrolytes make batteries safer and store more energy.
Lithium-ion batteries face problems like mining hurting the environment and low recycling rates. Better recycling methods are needed to fix these issues.
Key Materials in Li-ion Battery Components
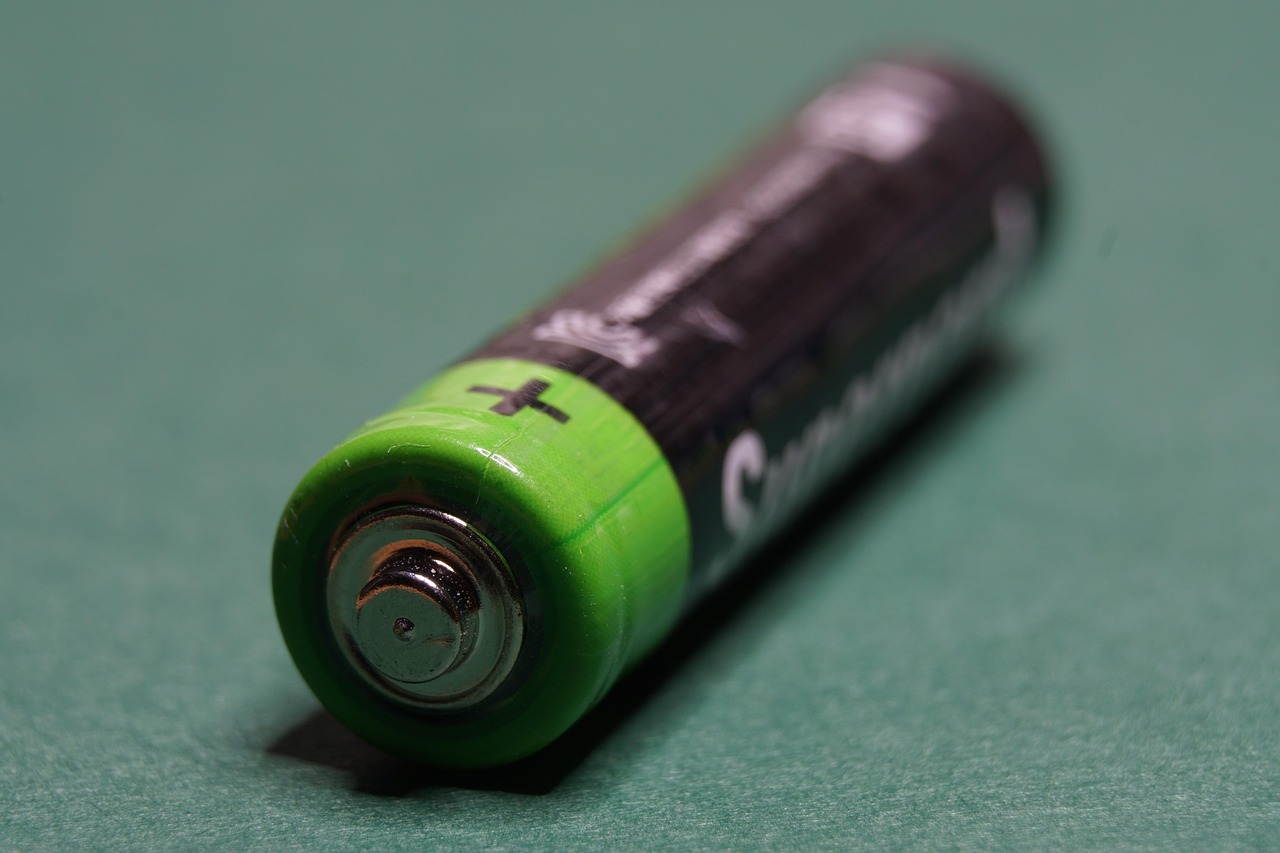
Lithium-ion batteries rely on carefully selected materials to achieve their exceptional performance. Each component—cathode, anode, electrolyte, and separator—uses specific materials that contribute to the battery’s efficiency, safety, and longevity. Below, you’ll find a detailed breakdown of these materials and their roles.
Cathode Materials
The cathode is a critical component that determines the energy density and voltage of lithium-ion batteries. Different cathode materials offer unique advantages:
Lithium cobalt oxide (LCO)
LCO is widely used in consumer electronics due to its high energy density. However, it has moderate safety and longevity, making it less suitable for applications requiring extended lifespans.
Lithium nickel manganese cobalt oxide (NMC)
NMC offers a balance of high energy density and moderate safety. It is commonly used in electric vehicles and energy storage systems. Innovations in ultra-high nickel layered oxides are further enhancing its performance.
Lithium iron phosphate (LFP)
LFP stands out for its high safety and longevity. It is increasingly popular in stationary energy storage and electric vehicles, particularly in China, due to its lower energy density but superior thermal stability.
Cathode Material | Energy Density | Safety | Longevity |
---|---|---|---|
High | Moderate | Moderate | |
Nickel Manganese Cobalt (NMC) | High | Moderate | Moderate |
Lithium Iron Phosphate (LFP) | Moderate | High | High |
Anode Materials
The anode stores lithium ions during charging and releases them during discharging. Its composition significantly impacts the battery’s energy density and charging speed.
Graphite
Graphite is the most commonly used anode material due to its stability and affordability. It provides reliable performance but has limitations in energy density.
Silicon-based anodes
Silicon-based anodes are emerging as a promising alternative. They can enhance energy density by 20-40% compared to graphite. Silicon also accommodates ten times more lithium ions per mass, enabling faster charging and higher power output.
Silicon-based anodes improve energy density by 20-40%.
Silicon can store ten times more lithium ions than graphite.
Electrolyte Materials
The electrolyte facilitates the movement of lithium ions between the cathode and anode. Its composition directly affects the battery’s conductivity and safety.
Liquid electrolytes (lithium salts in organic solvents)
Liquid electrolytes, typically composed of lithium salts like LiPF6 dissolved in organic solvents, are the most common choice. They provide excellent ion conductivity but require careful handling to ensure safety.
Solid-state electrolytes
Solid-state electrolytes are gaining attention for their potential to improve safety and energy density. Unlike liquid electrolytes, they are non-flammable and reduce the risk of thermal runaway.
A comprehensive dataset of 38 solvents and 14 lithium salts highlights the conductivity properties of various electrolyte compositions, ensuring reliable performance benchmarks.
Separator Materials
The separator in a lithium-ion battery plays a crucial role in maintaining safety and performance. It physically separates the cathode and anode while allowing lithium ions to pass through. The choice of separator material directly impacts the battery’s efficiency, durability, and safety.
Polyethylene (PE) and polypropylene (PP)
Polyethylene (PE) and polypropylene (PP) are the most commonly used separator materials in lithium-ion batteries. These polymers are lightweight, cost-effective, and provide excellent mechanical strength. PE separators are known for their thermal stability, which helps prevent overheating during operation. However, their low porosity can restrict ion transport, slightly reducing the battery’s overall performance.
Polypropylene (PP) separators, on the other hand, offer higher porosity compared to PE. This feature enhances ion flow, improving the battery’s conductivity and efficiency. Despite this advantage, PP separators are less flexible, which can pose challenges during the manufacturing process. Both materials are often used in combination to balance their strengths and limitations.
Tip: The combination of PE and PP layers in separators is a common strategy to optimize both thermal stability and ion transport.
Ceramic-coated separators
Ceramic-coated separators represent a significant advancement in battery technology. These separators combine the flexibility of polymer materials with a ceramic layer that enhances thermal stability and safety. The ceramic coating acts as a barrier against thermal runaway, reducing the risk of battery fires. This makes them an ideal choice for applications requiring high safety standards, such as electric vehicles and energy storage systems.
While ceramic-coated separators offer superior performance, they come with higher manufacturing costs. Their enhanced safety and durability, however, make them a worthwhile investment for critical applications.
The choice of separator material depends on the specific requirements of the battery, balancing cost, safety, and performance.
How Lithium-ion Batteries Are Made: Roles of Each Material
Understanding how lithium-ion batteries are made involves examining the roles of their key components. Each material contributes to the battery’s functionality, ensuring optimal performance and safety.
Cathode
Provides the source of lithium ions during discharge
The cathode serves as the primary source of lithium ions in lithium-ion batteries. During discharge, lithium ions move from the cathode to the anode through the electrolyte. This movement generates the electrical energy that powers your devices. The choice of cathode material directly impacts the battery’s energy density and voltage. For instance, materials like lithium cobalt oxide (LCO) and nickel manganese cobalt (NMC) offer high energy density, making them ideal for applications requiring compact, powerful batteries.
Determines the battery’s energy density and voltage
The cathode’s material composition dictates the battery’s energy density and voltage characteristics. For example, lithium iron phosphate (LFP) provides moderate energy density but excels in safety and longevity. In contrast, NMC and nickel cobalt aluminum (NCA) chemistries deliver higher energy density and voltage, making them suitable for electric vehicles. The table below highlights the performance metrics of various cathode chemistries:
Cathode Material | Energy Density | Voltage Characteristics |
---|---|---|
LFP | Moderate | Lower voltage |
NMC/NCA | High | Higher voltage |
LMFP | Bridging cost and density | Moderate to high voltage |
LNMO | Moderate | High voltage |
Anode
Stores lithium ions during charging
The anode acts as a storage unit for lithium ions during charging. Graphite, the most common anode material, allows for reversible lithium-ion storage, ensuring reliable battery performance. Silicon-based anodes are emerging as a superior alternative, offering higher energy density and faster charging capabilities.
Impacts battery lifespan and charging speed
The anode’s material significantly influences the battery’s lifespan and charging speed. Graphite provides stability and affordability, while silicon-based anodes enhance energy density by up to 40%. This improvement supports faster charging and longer-lasting batteries, making them ideal for high-performance applications.
Component | Role |
---|---|
Anode | Stores lithium ions during charging and releases them during discharging. Made from graphite, which allows for reversible storage. |
Electrolyte
Facilitates the movement of lithium ions between cathode and anode
The electrolyte serves as the medium for lithium-ion transport between the cathode and anode. State-of-the-art electrolytes consist of lithium salts dissolved in organic solvents. These solutions maximize ionic conductivity, ensuring efficient lithium-ion movement. The solvent mixture’s high electric permittivity and low viscosity further enhance ion transport.
Affects battery safety and performance
The electrolyte’s composition plays a crucial role in battery safety and performance. Proper dissociation of lithium salts in the non-aqueous medium ensures the availability of lithium ions for transport. This design minimizes resistance and maximizes efficiency, contributing to the overall functionality of lithium-ion batteries.
Tip: Electrolytes with non-flammable properties are gaining popularity for their ability to improve safety without compromising performance.
Electrolytes maximize ionic conductivity for efficient lithium-ion transport.
Organic solvents with low viscosity enhance ion mobility, reducing energy loss.
Separator
Prevents short circuits by separating cathode and anode
The separator in a lithium-ion battery acts as a physical barrier between the cathode and anode. This separation is essential to prevent direct contact between the two electrodes, which could lead to dangerous short circuits. Without this protective layer, the battery would fail to function safely, posing risks such as overheating or even thermal runaway.
You can think of the separator as the unsung hero of battery safety. It ensures that the electrodes remain isolated while still allowing lithium ions to pass through during charging and discharging. This dual functionality makes it a critical component in maintaining the battery’s reliability and safety.
Modern separators are engineered with advanced materials like polyethylene (PE) and polypropylene (PP), which provide excellent mechanical strength and thermal stability. These properties help the separator withstand the stresses of repeated charging cycles, ensuring long-term durability.
Note: A high-quality separator is vital for preventing short circuits, especially in high-performance applications like electric vehicles and energy storage systems.
Ensures ion flow while maintaining structural integrity
While the separator prevents short circuits, it also plays an active role in the battery’s performance. It facilitates the movement of lithium ions between the cathode and anode, a process that is crucial for the battery’s energy transfer. This ion flow enables the charging and discharging cycles that power your devices.
The separator’s porous structure allows ions to pass through while maintaining its mechanical integrity. This balance ensures that the battery operates efficiently without compromising safety. Advanced separators, such as ceramic-coated ones, enhance this functionality by providing additional thermal stability and resistance to mechanical stress.
The separator’s ability to support ion flow while maintaining its structure directly impacts the battery’s efficiency and lifespan. By choosing the right separator material, manufacturers can optimize both safety and performance, ensuring that the battery meets the demands of modern applications.
Sustainability Challenges in Lithium-ion Battery Materials
Environmental Impact of Mining
Lithium extraction and water usage
The extraction of lithium, a key material in lithium-ion batteries, poses significant environmental challenges. Hard-rock lithium mining generates up to 2.5 times more carbon emissions than brine-based methods, depending on the energy sources used. Brine extraction, while less carbon-intensive, consumes substantial amounts of water. This process often withdraws water that is not returned to its original source, impacting local ecosystems. In regions with limited freshwater availability, such as the Atacama Desert, lithium extraction exacerbates water scarcity, threatening biodiversity and local communities.
Evidence Type | Measurement Details |
---|---|
Water Consumption | Withdrawn water not returned to the source, including city water and embodied water in electricity. |
CO2-eq Emissions | Up to 2.5 times higher for hard-rock mining compared to brine extraction. |
Cobalt mining and ethical concerns
Cobalt, another critical material, presents ethical and environmental challenges. Approximately 60% of the global cobalt supply comes from the Democratic Republic of Congo (DRC), where mining often involves child labor and unsafe working conditions. These practices raise significant ethical concerns. Additionally, cobalt mining contributes to deforestation and soil degradation, further harming the environment.
Recycling and End-of-Life Management
Challenges in recycling lithium-ion batteries
Battery recycling remains a significant hurdle. Globally, only 2% to 47% of lithium-ion batteries are recycled, compared to a 99% recycling rate for lead-acid batteries. The low recycling rate stems from technical challenges, high costs, and the complexity of recovering materials like lithium, cobalt, and nickel.
Current recycling technologies and their limitations
Current recycling methods, such as hydrometallurgy and pyrometallurgy, offer varying levels of efficiency. Hydrometallurgy provides better energy efficiency but generates secondary waste. Pyrometallurgy, while simpler, has higher environmental impacts. Despite these limitations, recycling can reduce greenhouse gas emissions and water consumption by at least 58% compared to traditional mining.
Supply Chain and Resource Scarcity
Dependence on limited resources like cobalt and nickel
The supply chain for lithium-ion batteries heavily depends on scarce materials like cobalt and nickel. For instance, 20% of the global high-grade nickel supply comes from Russia, creating vulnerabilities in the supply chain. By 2030, cobalt production is expected to face a 20% shortfall, further straining resources.
Geopolitical issues in material sourcing
Geopolitical factors also complicate material sourcing. The DRC supplies 60% of the world’s cobalt, making the supply chain susceptible to political instability. Additionally, the price of nickel spiked by 250% in early 2022, highlighting the volatility of these critical materials.
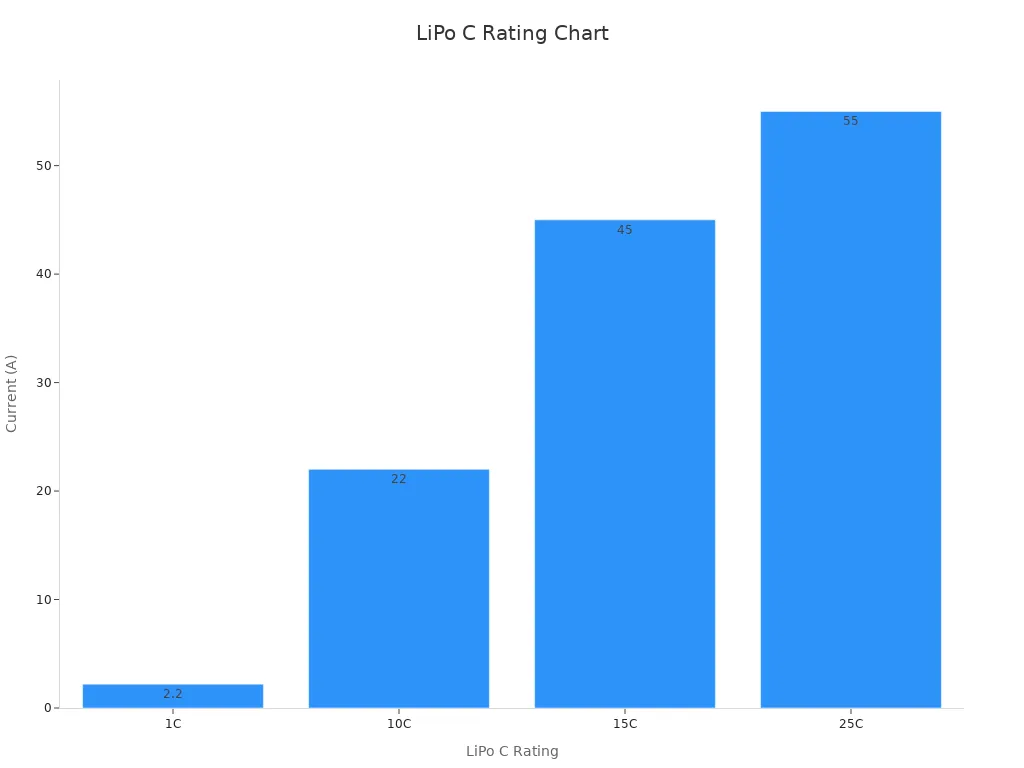
Note: Addressing these sustainability challenges requires advancements in recycling technologies and the development of alternative materials.
Emerging Alternatives and Innovations in Li-ion Battery Production
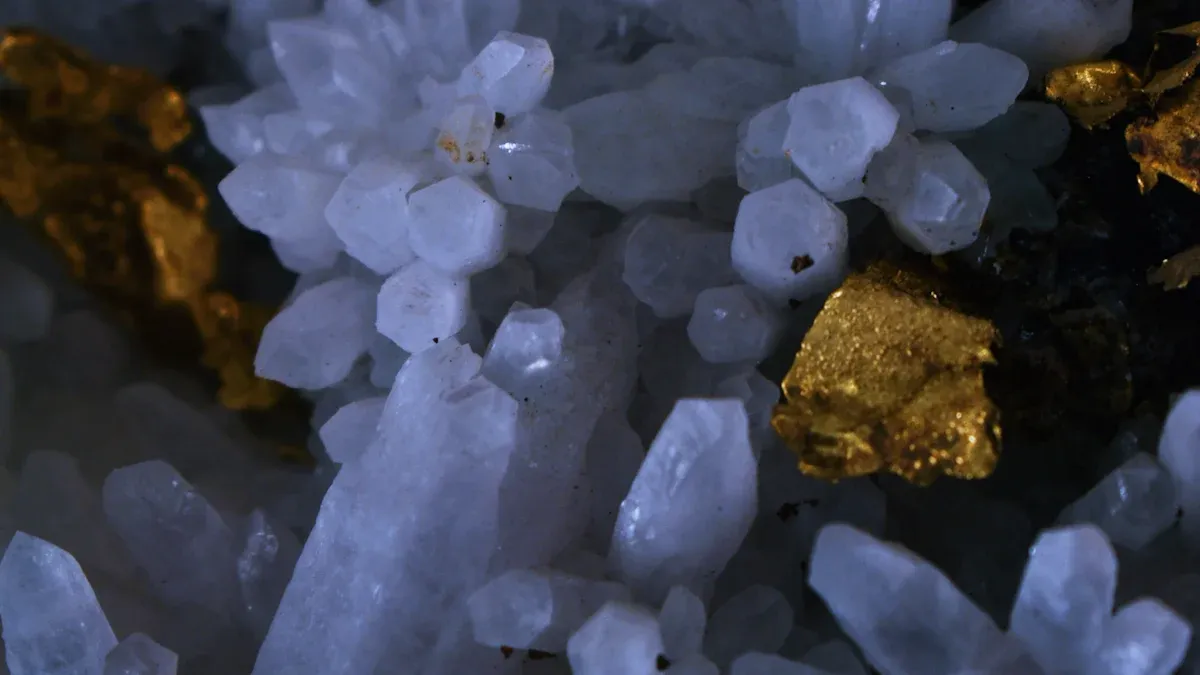
The rapid evolution of lithium-ion battery technology has led to groundbreaking innovations in materials and manufacturing processes. These advancements aim to improve energy density, safety, and sustainability, addressing the growing demand for efficient energy storage in applications like electric car batteries.
Alternative Cathode Materials
Cobalt-free cathodes
Cobalt-free cathodes are gaining traction as a sustainable alternative in battery manufacturing. By eliminating cobalt, these cathodes reduce environmental and ethical concerns associated with mining. They also lower production costs, making them an attractive option for large-scale applications like electric car batteries. Researchers are exploring manganese-rich chemistries to replace cobalt while maintaining high energy density and safety.
High-nickel and manganese-rich chemistries
High-nickel cathodes are revolutionizing battery chemistries by offering superior energy density. These materials reduce cobalt dependency while enhancing performance. Manganese-rich chemistries, on the other hand, provide a cost-effective solution with improved thermal stability. Both options are pivotal in advancing battery cell manufacturing for next-generation applications.
Next-Generation Anodes
Silicon anodes for higher energy density
Silicon anodes represent a significant leap in battery electrode materials. They can store 20-40% more energy than traditional graphite anodes, enabling longer-lasting batteries. Companies like Sila Nanotechnologies are pioneering this technology, which promises to enhance the performance of li-ion batteries. However, challenges like swelling during charging cycles require further refinement to ensure reliability.
Lithium metal anodes for solid-state batteries
Lithium metal anodes are at the forefront of solid-state battery innovation. These anodes achieve energy densities exceeding 400 Wh/kg, making them ideal for high-performance applications. QuantumScape is leading efforts to commercialize this technology, which eliminates flammable liquid electrolytes, enhancing safety and efficiency.
Battery Type | Key Innovations | Energy Density Improvement |
---|---|---|
Silicon Anodes | Boost energy density by 20-40% over graphite | 20-40% |
Lithium-Metal Anodes | Solid-state design with energy densities of 400 Wh/kg or more | High |
Advanced Electrolytes and Separators
Non-flammable electrolytes for improved safety
Non-flammable electrolytes are transforming battery manufacturing by addressing fire safety concerns. These formulations have a flash point exceeding 70°C and a self-extinguishing time 25 times better than standard electrolytes. This innovation supports fast charging and enhances the safety of li-ion batteries, particularly in e-mobility applications.
Note: AI-driven material discovery has accelerated the development of safer electrolytes, identifying millions of potential formulations in record time.
Innovations in solid-state battery separators
Solid-state separators are redefining battery chemistries by replacing traditional liquid electrolytes. These separators improve ionic conductivity and eliminate flammability risks, making them a cornerstone of next-generation battery cell manufacturing. Their robust design ensures structural integrity, even under extreme conditions, paving the way for safer and more efficient batteries.
The integration of advanced electrolytes and separators is crucial for achieving the performance and safety standards required in modern energy storage systems.
Recycling and Circular Economy
New recycling methods for higher material recovery
Advancements in battery recycling are transforming how valuable materials are recovered from lithium-ion batteries. New methods now achieve higher recovery rates for critical materials like nickel, cobalt, and lithium. For example, modern processes can recover up to 95% of nickel and cobalt and 92% of lithium, ensuring minimal waste.
Material | Recovery Rate |
---|---|
Ni | 95% |
Co | 95% |
Li | 92% |
These innovations not only improve recovery rates but also enhance the purity of recycled materials. Efficient recycling methods reduce the need for raw material extraction, lowering environmental impact. Additionally, design improvements in battery manufacturing simplify disassembly, making recycling more efficient. This approach increases profitability for recycling facilities while supporting a sustainable supply chain.
Recycling technologies now focus on maximizing material purity.
Improved designs allow easier battery disassembly.
Enhanced processes boost both efficiency and profitability.
By adopting these methods, you contribute to a circular economy where resources are reused instead of discarded.
Closed-loop systems for sustainable battery production
Closed-loop systems represent a significant step toward sustainable battery production. These systems recycle materials from end-of-life batteries and reintegrate them into new battery manufacturing. This approach minimizes waste and reduces dependency on mining for raw materials.
For instance, recovered nickel, cobalt, and lithium can be directly reused in producing new batteries, maintaining high performance without compromising quality. Closed-loop systems also reduce greenhouse gas emissions by eliminating the need for energy-intensive mining processes.
Tip: Supporting companies that implement closed-loop systems helps promote sustainability in the battery industry.
Recycling and second-life applications further enhance the value of these systems. Batteries that no longer meet performance standards for their original use can serve secondary purposes, such as energy storage in renewable energy systems. This practice extends the lifecycle of batteries, reducing waste and conserving resources.
By embracing closed-loop systems, you help create a sustainable future where battery recycling and second-life applications become the norm.
Lithium-ion batteries depend on a precise combination of materials to deliver exceptional performance and functionality. These materials, while enabling the widespread adoption of lithium-ion batteries, pose challenges related to sustainability. Scaling up production to meet the growing demand for electric vehicles and renewable energy storage highlights the need for sustainable solutions. Recycling processes, which can recover up to 95% of valuable materials like nickel and cobalt, offer a promising path toward reducing environmental impacts.
By 2030, the recycling industry could recover between 400,000 and 1 million tons of materials from spent batteries, creating a market opportunity worth approximately $6 billion.
Innovations in battery materials and recycling technologies are paving the way for a more sustainable future. Large Power, with its expertise in custom lithium battery solutions, continues to lead the charge in delivering advanced and sustainable battery technologies for diverse applications.
FAQ
What makes lithium-ion batteries so popular?
Lithium-ion batteries offer high energy density, lightweight design, and long lifespan. These features make them ideal for powering devices like smartphones, laptops, and electric vehicles. Their ability to recharge quickly and maintain performance over many cycles further enhances their appeal.
Are lithium-ion batteries safe to use?
Yes, lithium-ion batteries are safe when used correctly. Manufacturers incorporate safety features like thermal management systems and separators to prevent overheating or short circuits. However, you should avoid exposing them to extreme temperatures or physical damage to ensure optimal safety.
How can you extend the lifespan of a lithium-ion battery?
To extend battery life, avoid overcharging or fully discharging it. Keep the battery at a moderate temperature and use the recommended charger. Partial charging cycles and proper storage when not in use also help maintain its performance.
Can lithium-ion batteries be recycled?
Yes, lithium-ion batteries can be recycled to recover valuable materials like lithium, cobalt, and nickel. Recycling reduces environmental impact and conserves resources. However, recycling rates remain low due to technical challenges, making proper disposal and recycling initiatives essential.
What are the main applications of lithium-ion batteries?
Lithium-ion batteries power a wide range of devices, including smartphones, laptops, and electric vehicles. They are also used in renewable energy storage systems, medical devices, and industrial machinery due to their efficiency and reliability.
Tip: Choose a battery designed for your specific application to maximize performance and safety.