Contents
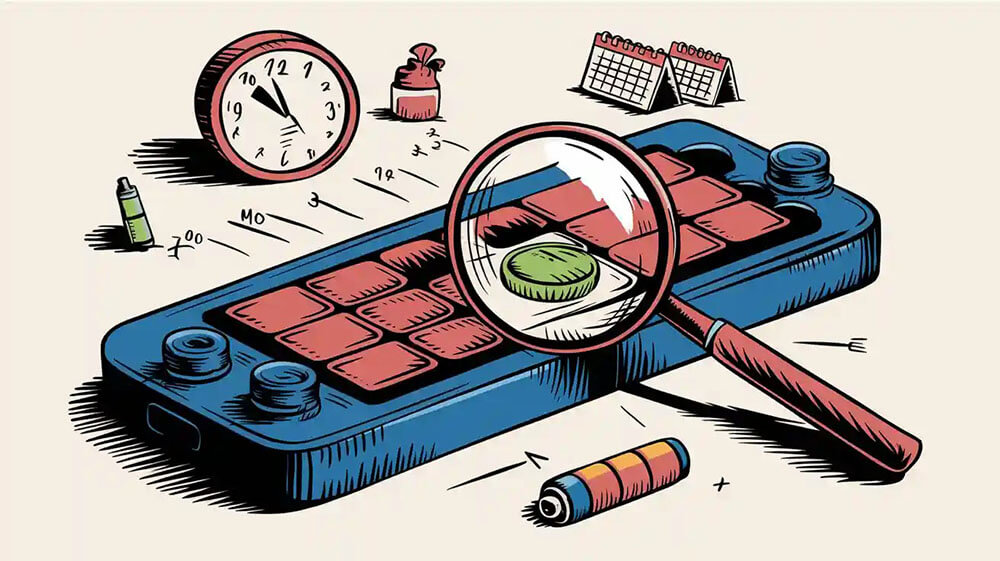
Battery aging refers to the gradual decline in a battery’s performance and capacity over time. This process impacts industries that depend on lithium-ion battery packs, such as medical equipment, energy storage systems, and consumer electronics. For instance, studies show that as batteries age, their capacity significantly decreases, which is a critical indicator of health. Batteries often fail due to capacity fade rather than increased internal resistance or low cold cranking amps (CCA). Understanding the aging of batteries helps you optimize performance and extend the lifespan of your battery systems.
Explore sustainable battery solutions here.
Key Takeaways
Check battery capacity often. Finding issues early keeps it working well.
Charge batteries smartly. Don’t overcharge or let them drain fully. This lowers stress and makes them last longer.
Keep batteries at good temperatures. Right temperatures stop fast aging and keep them safe.
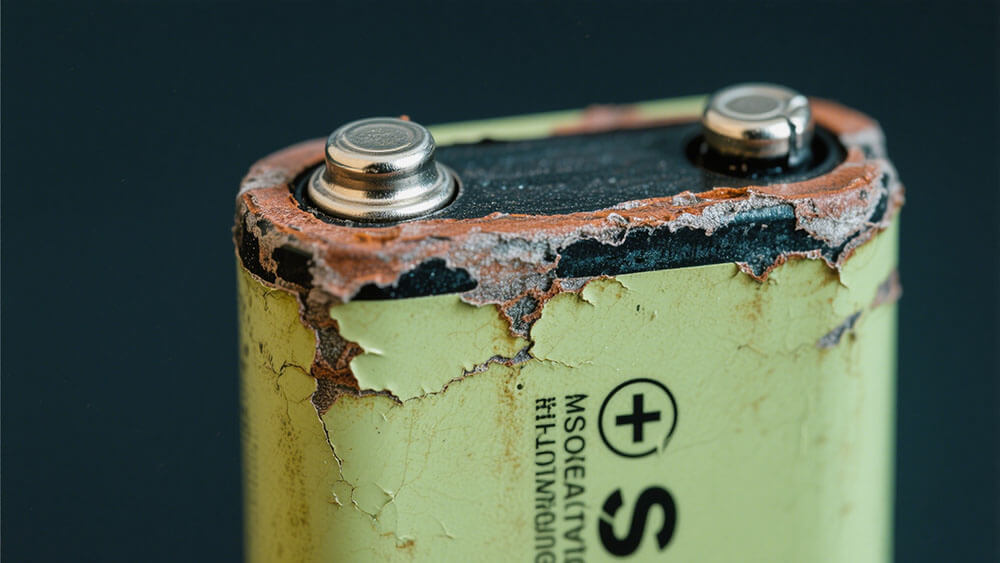
Part 1: The Science Behind the Aging of Batteries
1.1 What is battery aging, and why does it occur?
Battery aging refers to the gradual decline in performance and efficiency of batteries over time. This process occurs due to chemical, physical, and environmental factors that degrade the internal components. For lithium-ion batteries, aging is primarily caused by the breakdown of active materials, electrolyte decomposition, and the formation of a solid electrolyte interface (SEI) layer. These changes reduce the battery’s ability to store and deliver energy effectively.
A four-year study on LiFePO4 batteries revealed that calendar aging significantly contributes to capacity loss. Batteries stored at higher temperatures experienced faster degradation, while lower temperatures slowed the process. For instance, at 25°C and 50% state of charge (SOC), the model predicted a lifespan of 23.8 years before the battery capacity dropped to 80%.
1.2 Key mechanisms: calendar aging vs. cyclic aging
Battery aging occurs through two primary mechanisms: calendar aging and cyclic aging. Calendar aging happens when a battery degrades over time, even without use. Factors like high temperatures and prolonged storage at full charge accelerate this process. On the other hand, cyclic aging results from repeated charge and discharge cycles. High charge rates, deep discharges, and fast charging can intensify this type of aging.
Type of Aging | Key Factors Influencing Aging |
---|---|
Calendar Aging | Strongly affected by temperature; significant capacity loss over time, especially at higher temperatures (e.g., 45°C). |
Cyclic Aging | Influenced by cycling conditions and temperature, with distinct modeling approaches for capacity loss. |
1.3 Impact on capacity, internal resistance, and battery life cycle
As batteries age, their capacity diminishes, internal resistance increases, and the overall life cycle shortens. A dataset containing over 3 billion data points from lithium-ion cells demonstrated that both calendar and cyclic aging contribute to these changes. Increased internal resistance leads to inefficiency and heat generation, while reduced capacity limits the battery’s runtime. Dynamic discharge profiles, however, have shown to enhance battery lifetime by up to 38% compared to constant current discharge.
Understanding these impacts is crucial for optimizing battery energy storage systems and extending the lifespan of lithium-ion batteries. By addressing the signs of aging early, you can mitigate capacity loss and maintain performance.
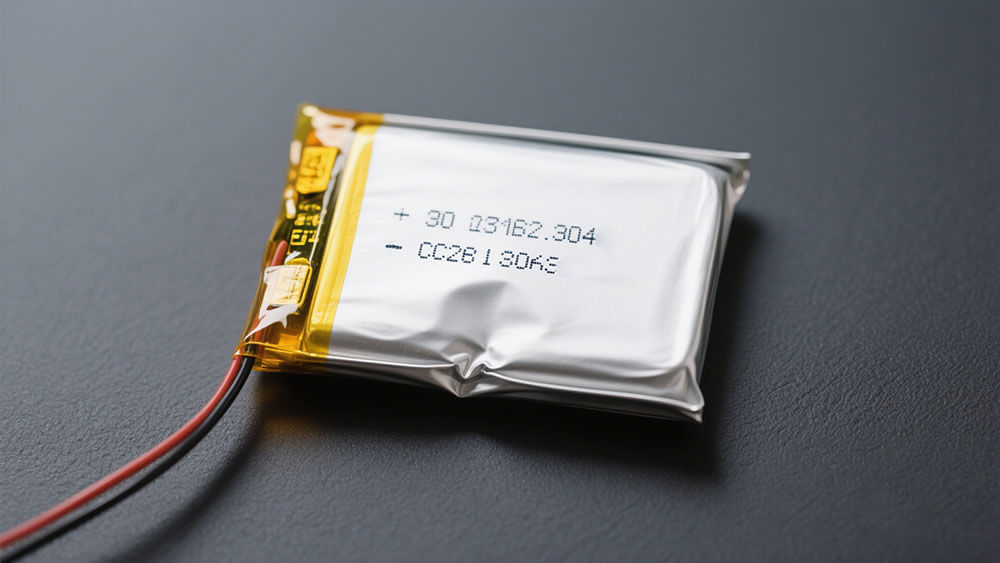
Part 2: Identifying Signs of Battery Aging
As batteries age, their performance declines, often in noticeable ways. Recognizing these signs early can help you take proactive measures to maintain efficiency and extend the lifespan of your battery systems.
2.1 Reduced capacity and shorter runtime
One of the most apparent signs of battery aging is reduced capacity. You may notice that your device no longer lasts as long on a full charge as it once did. This decline occurs because the battery’s ability to store energy diminishes over time. Additionally, you might experience inconsistent battery levels, where the percentage drops suddenly or fluctuates. In severe cases, the battery may swell or show physical damage, indicating advanced aging. These symptoms highlight the importance of monitoring capacity loss to ensure optimal performance.
2.2 Increased charging times and inefficiency
Aging batteries often take longer to charge fully. This inefficiency stems from the degradation of internal components, which hampers the battery’s ability to accept and store energy efficiently. You might also notice unexpected shutdowns, even when the battery appears to have a charge. Such behavior signals that the battery struggles to maintain a stable voltage, further emphasizing the impact of lithium battery aging on performance.
2.3 Higher internal resistance and heat generation
As batteries age, their internal resistance increases due to changes like the growth of the solid electrolyte interphase (SEI) layer and lithium plating. This rise in resistance not only reduces efficiency but also generates more heat during use and charging. Elevated temperatures can accelerate the aging of batteries, creating a cycle of degradation. For lithium-ion batteries, managing heat is crucial to maintaining safety and extending their life cycle.
By identifying these signs, you can better understand the aging process and take steps to mitigate its effects. Whether you’re managing battery energy storage systems or everyday devices, staying vigilant ensures reliable performance.
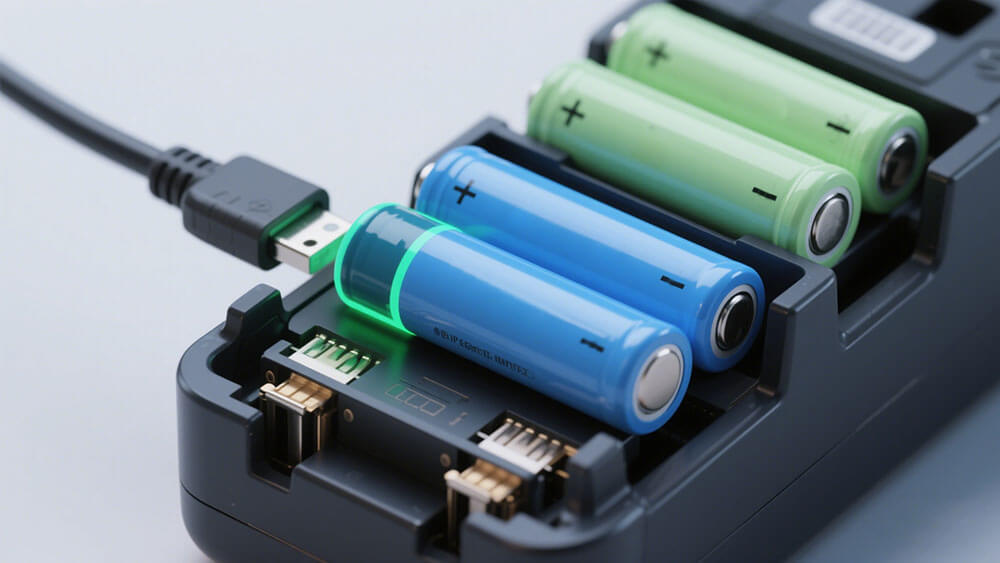
Part 3: Factors Accelerating Lithium Battery Aging
3.1 High operating temperatures and thermal stress
Operating lithium-ion batteries at high temperatures significantly accelerates their aging process. Elevated temperatures speed up chemical reactions within the battery, leading to faster degradation of internal components. Prolonged exposure to heat can compromise battery longevity and, in extreme cases, result in thermal runaway, posing safety risks. For instance:
High temperatures increase the rate of electrolyte decomposition, causing capacity loss.
Thermal stress can damage the solid electrolyte interphase (SEI) layer, reducing efficiency.
Excessive heat may lead to swelling or leakage, further shortening the battery’s lifespan.
To mitigate these effects, you should implement robust thermal management systems in battery energy storage applications. These systems help maintain optimal operating temperatures, ensuring safety and extending battery life.
3.2 Overcharging, overvoltage, and deep discharges
Improper charging practices, such as overcharging or deep discharges, can severely impact battery aging. Overcharging raises the battery’s temperature, increasing the risk of swelling or even fire. Deep discharges, on the other hand, strain the battery’s active materials, leading to irreversible damage. For example:
A vehicle with a full State of Health (SOH) offers a range of 150 km. At 80% SOH, this range drops to 120 km, highlighting the impact of poor charging habits on usability.
Overvoltage conditions accelerate the breakdown of internal components, reducing the battery’s lifespan.
Adopting smart battery management systems can prevent these issues by regulating voltage and charge levels, ensuring safe and efficient operation.
3.3 High charge/discharge rates and fast charging
Frequent high charge and discharge cycles can accelerate lithium battery aging. Fast charging generates heat and increases internal resistance, which reduces efficiency and shortens the battery’s life. Key performance metrics affected include:
Metric | Description |
---|---|
Capacity fade | Reduction in the battery’s ability to store energy, leading to shorter operational times. |
Increased internal resistance | Higher resistance affects power delivery efficiency, resulting in slower charging and increased heat. |
State of Health (SOH) | Represents the overall condition of the battery relative to its original capacity, indicating remaining lifespan. |
To minimize these effects, you should avoid frequent fast charging and maintain moderate charge/discharge rates. This approach helps preserve the battery’s capacity and performance over time.
3.4 Poor storage conditions and prolonged inactivity
Storing batteries in suboptimal conditions can accelerate aging. High humidity, extreme temperatures, and prolonged inactivity degrade the battery’s internal structure. For instance, lithium-ion batteries stored at full charge in hot environments experience faster capacity loss. To prevent this:
Store batteries at 50% charge in a cool, dry place.
Avoid leaving batteries unused for extended periods, as inactivity can lead to self-discharge and reduced performance.
Proper storage practices and regular maintenance ensure the longevity of your battery systems, reducing the need for frequent replacements.
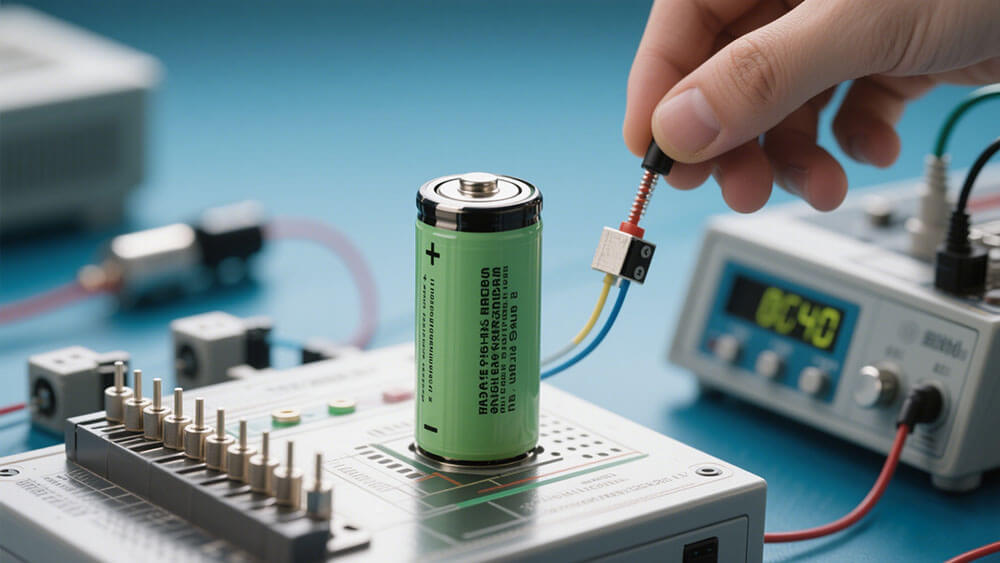
Part 4: Practical Strategies to Slow Down Battery Aging
4.1 Temperature management for lithium-ion batteries
Effective temperature management plays a critical role in slowing down battery aging. Lithium-ion batteries operate best within a specific temperature range, typically between 20°C and 25°C. Exceeding this range accelerates chemical reactions that degrade internal components, leading to capacity loss and reduced lifespan.
An experimental study on thermal management techniques revealed that active cooling methods, such as liquid cooling systems, effectively reduce heat during deep charge and discharge cycles. Passive methods, like heat sinks, work well under moderate conditions. Hybrid strategies, combining active and passive cooling, provide an optimal balance between energy efficiency and thermal performance. These approaches are particularly beneficial for battery energy storage systems operating under varying demands.
Maintaining stable temperatures not only extends battery life but also ensures safety by preventing thermal runaway. Implementing robust cooling systems is essential for industries relying on large-scale battery packs, such as renewable energy storage and electric vehicles.
4.2 Optimizing charging practices and avoiding extremes
Charging practices significantly impact lithium battery aging. Overcharging or deep discharges strain the battery’s active materials, accelerating degradation. To optimize charging:
Avoid charging beyond 80% state of charge (SOC), as studies show this reduces stress on the battery and extends its lifespan.
Minimize deep discharges by maintaining the charge level between 20% and 80%.
Use chargers with built-in voltage regulation to prevent overvoltage conditions.
These practices not only preserve battery health but also improve efficiency during charge and discharge cycles. Educating users on optimal charging habits is crucial for industries like medical equipment, where reliability is paramount.
4.3 Implementing smart battery management systems (BMS)
Smart battery management systems (BMS) enhance battery longevity by monitoring and controlling critical parameters. These systems diagnose battery health, predict performance, and optimize usage through advanced algorithms. For example:
Metric/Method | Description |
---|---|
Diagnosis | Tools to assess battery health using rapid, scalable measurements. |
Prediction | Anticipates future performance using lab data and online diagnostics. |
Optimization | Extends battery life while meeting performance metrics through predictive models and control algorithms. |
By integrating a BMS, you can achieve up to a 30% improvement in battery lifetime. This reduces the total cost of ownership for battery energy storage systems, making it a valuable investment for industrial applications.
4.4 Tailored approaches for medical equipments, energy storage systems, and industrial applications
Different applications require customized strategies to combat battery aging. For medical equipment, maintaining stable temperatures and avoiding deep discharges ensures reliability during critical operations. In energy storage systems, smart BMS and hybrid cooling methods optimize performance and extend battery lifespans. Case studies, such as the Moura project in Brazil, demonstrate how integrating solar panels with battery energy storage systems can reduce carbon emissions and improve efficiency.
Industrial applications benefit from advanced storage solutions like those implemented by Exide Group in Portugal. These systems achieve a 20% reduction in carbon emissions while extending battery lifetimes from 10 to 20 years under optimal conditions. Tailored strategies not only enhance performance but also contribute to sustainability goals.
For more insights into sustainable battery solutions, visit Sustainability at Large Power.
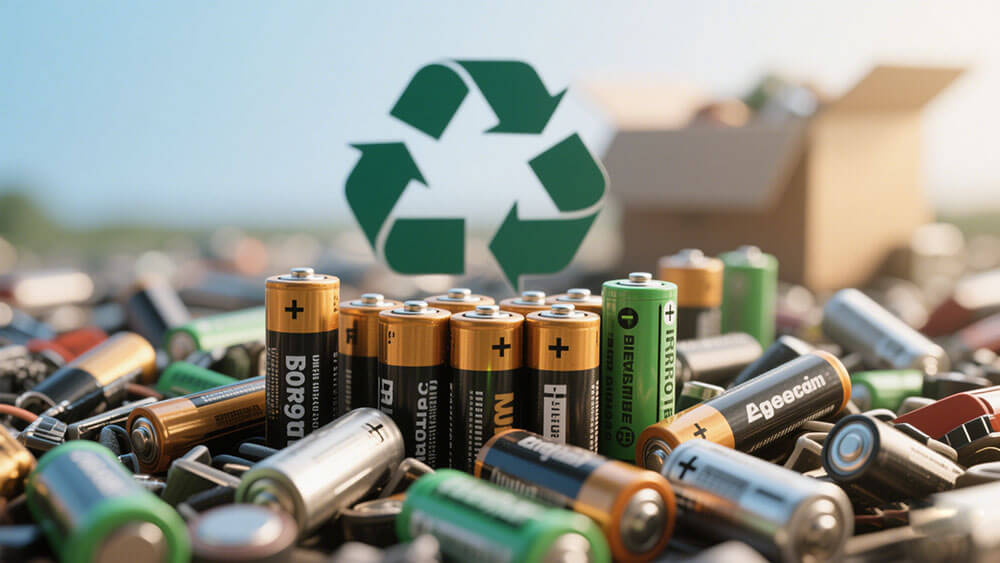
Part 5: Can Aged Lithium-Ion Batteries Still Be Used?
5.1 Repurposing aged batteries for secondary applications
Aged lithium-ion batteries often retain sufficient capacity for secondary applications, even after their primary use. For example, old electric vehicle (EV) batteries can support the electricity grid by supplying stored energy during peak demand. Nissan has successfully repurposed these batteries to power streetlights using solar energy and provide backup power for train signals. In France, a project utilizes retired EV batteries to power a data center, showcasing their versatility in lower-power applications.
These examples highlight the feasibility of repurposing aged batteries to extend their utility and reduce waste. By leveraging their remaining capacity, you can contribute to sustainable energy solutions while optimizing battery performance in secondary roles.
5.2 Evaluating safety and performance for continued use
Before reusing aged batteries, evaluating their safety and performance is essential. Comprehensive testing ensures that batteries meet safety standards and perform reliably throughout their extended lifecycle. Testing identifies vulnerabilities, such as risks of thermal runaway or short circuits, and ensures compliance with international safety standards.
Key metrics like the state of health (SOH) help assess battery degradation. SOH measures internal resistance and capacity compared to a new battery. Accurate SOH estimation enables the development of advanced battery management systems (BMS) to monitor and optimize battery lifespan. These evaluations ensure that aged batteries remain safe and efficient for continued use.
5.3 Recycling and disposal of lithium-ion batteries
Recycling lithium-ion batteries addresses environmental concerns and supports the clean energy transition. Proper recycling prevents issues caused by improper disposal and recovers valuable minerals like lithium, cobalt, and nickel. These materials are essential for producing new batteries and meeting the growing demand for renewable energy technologies.
Aspect | Details |
---|---|
Market Size | |
Trends | Adoption of recycling technologies across regions. |
Competitive Landscape | Strategies of key players in the recycling industry. |
Recycling also reduces reliance on conflict minerals, promoting ethical sourcing practices. To learn more about conflict mineral statements, visit Conflict Minerals Statement. By prioritizing recycling, you can support sustainability goals and reduce the environmental impact of battery disposal.
Understanding battery aging is essential for optimizing performance, reducing costs, and achieving sustainability. Batteries play a critical role in clean energy transitions and are among the most expensive components in energy systems. You can extend battery life by managing temperature, adopting smart charging practices, and repurposing aged batteries. Explore sustainable battery solutions for tailored strategies.
FAQ
1. How can you extend the battery life cycle of lithium-ion batteries?
You can extend the battery life cycle by maintaining optimal temperatures, avoiding deep discharges, and using smart charging practices. These strategies reduce stress on internal components.
2. What is the typical cycle life of a lithium-ion battery?
The cycle life of a lithium-ion battery ranges from 500 to 2,000 cycles. This depends on factors like battery chemistries, operating conditions, charging habits, and temperature management.
3. Why does battery life decrease over time?
Battery life decreases due to chemical reactions, physical wear, and environmental factors. These cause capacity loss, increased resistance, and reduced efficiency during the battery’s life cycle.
For custom battery solutions tailored to your needs, explore Custom Battery Solutions.