Contents
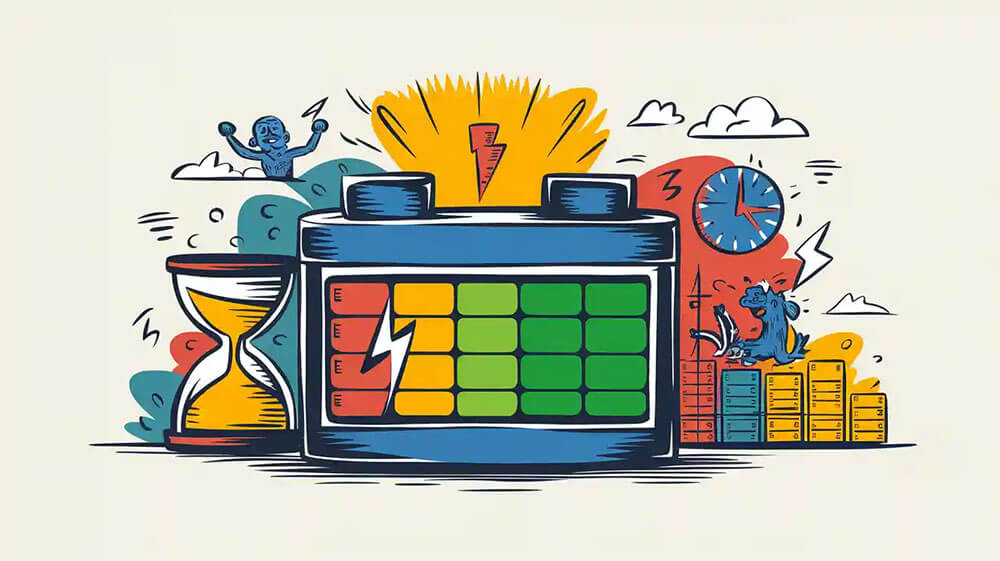
Battery cycle life refers to the number of complete charge and discharge cycles a battery can undergo before its capacity drops below 80% of its original value. This metric plays a critical role in industrial and energy storage applications. For instance:
- A battery with a cycle life of 1,000 can sustain 1,000 cycles before its capacity diminishes significantly.
- Batteries typically reach the end of their useful life when their capacity falls to around 80%.
A longer cycle life ensures fewer replacements, reducing costs and enhancing reliability. In sectors like solar energy storage and medical devices, the longevity of a battery directly impacts operational efficiency and cost-effectiveness. Understanding battery cycle life enables you to select and manage batteries that align with your needs.
Key Takeaways
- Battery cycle life shows how many times a battery can charge and discharge before it holds less than 80% power. Knowing this helps you pick the best battery for your use.
- A longer cycle life means you replace batteries less often. This saves money and creates less trash. For example, LiFePO4 batteries can last up to 15 years. They are a good choice for storing energy.
- Charging the right way and keeping batteries cool can make them last longer. Keep batteries half-charged and avoid very hot or cold places to help them work better for more time.
Part 1: What is Battery Cycle Life?
1.1 Definition and Explanation of Battery Cycle Life
Battery cycle life refers to the number of complete charge and discharge cycles a battery can undergo before its capacity drops below 80% of its original value. This metric is critical for evaluating the longevity and reliability of batteries in industrial applications.
- A battery’s life cycle ends when it can no longer hold a charge effectively, typically when its capacity falls below 80%.
- Depth of Discharge (DoD) plays a significant role in determining cycle life. It measures the percentage of the battery’s capacity used during each cycle. Batteries with lower DoD generally last longer.
For example, lithium-ion batteries often achieve 300-2,000 cycles, while lead-acid batteries typically endure 200-300 cycles. Understanding these parameters helps you optimize battery performance and plan for replacements effectively.
1.2 How to Calculate Battery Life Cycle
To calculate battery life cycle, you need to consider the Depth of Discharge (DoD) and the total capacity utilized during each cycle. The formula involves dividing the total capacity delivered by the end-of-life threshold. For instance:
Battery Life Cycle = Total Delivered Capacity ÷ End-of-Life Threshold
This calculation provides a rough estimate of the number of cycles a battery can complete before degradation. Monitoring DoD and charging practices can help you extend the battery’s usable life.
1.3 Comparison of Battery Chemistries and Their Cycle Life
Different battery chemistries exhibit varying cycle life performances. Below is a comparison of common chemistries:
Battery Chemistry | Cycle Life (Cycles) |
---|---|
LiFePO4 | 2,000 – 5,000 |
NCM | 1,000 – 2,000 |
LMO | 300 – 700 |
LCO | 500 – 1,000 |
LTO | Up to 10,000 |
According to the 2024 Battery Technology White Paper published by the U.S. Department of Energy (DOE), LiFePO4 batteries have become the preferred choice for energy storage systems due to their high cycle life and thermal stability.
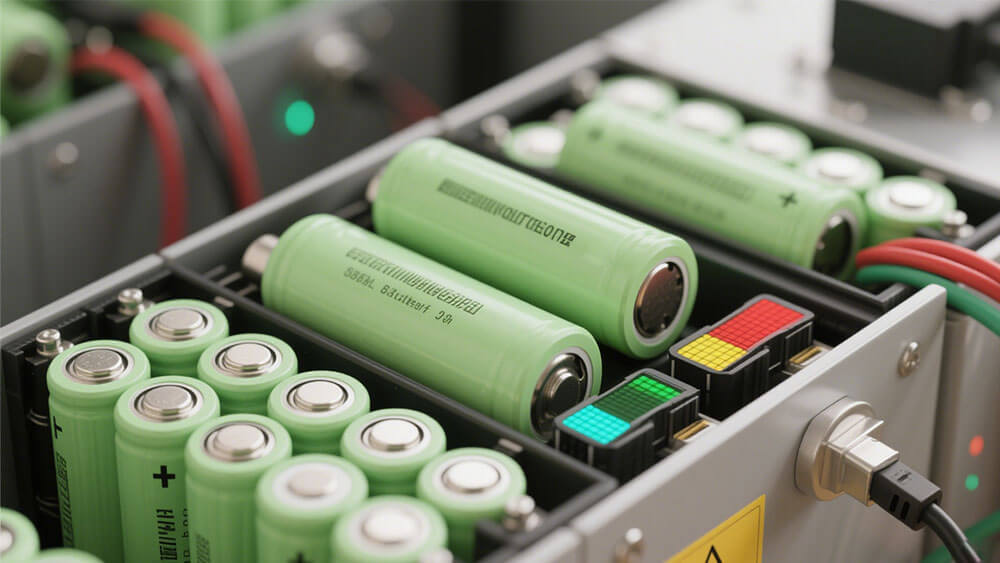
Part 2: Why Battery Cycle Life Matters
2.1 Impact on Battery Longevity and Replacement Costs
Battery cycle life plays a pivotal role in determining battery longevity and the frequency of replacements. A longer cycle life directly translates to fewer replacements, reducing operational costs and downtime. For instance, Tesla’s Powerwall home energy storage system utilizes LiFePO4 batteries with a cycle life of 5,000 cycles. Assuming daily charge/discharge cycles, this translates to over 13 years of service, drastically reducing replacement frequency and costs for users. This longevity makes them a cost-effective choice for applications like solar energy storage and electric vehicles.
📊 Key Benefits of Longer Cycle Life:
Benefit Description Cost Savings Fewer replacements lower operational expenses. Reliability Extended cycle life ensures dependable performance in critical systems. Sustainability Longer-lasting batteries reduce waste and environmental impact.
Maximizing battery life cycle is essential for cost efficiency. Batteries with shorter cycle lives require frequent replacements, increasing both costs and environmental impact. By selecting batteries with higher cycle life, you can enhance battery health and reduce long-term expenses.
2.2 Role in Performance and Reliability of Battery Packs
The performance and reliability of battery packs heavily depend on their cycle life. Batteries with extended cycle life maintain consistent battery capacity over time, ensuring stable performance in demanding applications. For example, in electric vehicles, a reliable battery lifespan ensures optimal driving range and reduces the need for frequent replacements.
Battery chemistry significantly influences cycle life and, consequently, battery performance. LiFePO4 batteries, for instance, offer superior reliability due to their high cycle life and stable discharge rates. In contrast, chemistries like LCO or LMO, with shorter cycle lives, may degrade faster, impacting overall reliability. Factors such as charging rates and temperature management also play a critical role in preserving battery health and ensuring consistent performance.
In applications like solar energy storage, batteries with longer cycle life provide uninterrupted energy supply over years, enhancing system reliability. By prioritizing batteries with extended cycle life, you can achieve better performance, reduced maintenance, and greater operational efficiency.
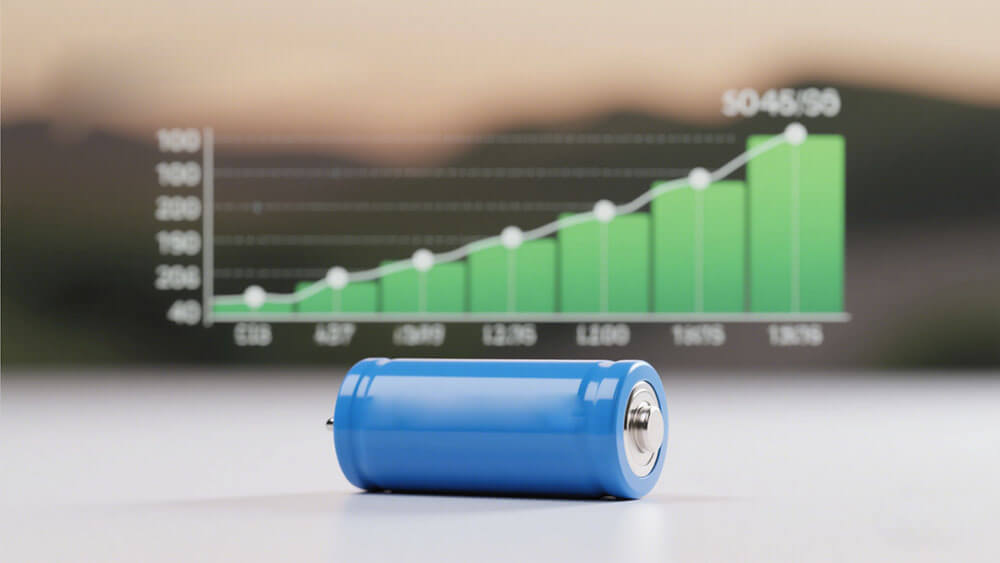
Part 3: Factors Affecting Battery Cycle Life
3.1 Depth of Discharge (DoD) and Its Impact
Depth of Discharge (DoD) significantly influences battery cycle life. John Smith, Director of Battery Technology at Tesla, notes: ‘Depth of Discharge (DoD) is a critical factor influencing lithium-ion battery longevity. Maintaining DoD below 50% can significantly extend battery lifespan.’ It measures how much of a battery’s capacity is used during each cycle. Lower DoD levels generally extend the lifespan of rechargeable batteries. For instance:
- Cycling lead-acid batteries to 50% DoD instead of 80% can double their lifespan.
- Reducing DoD to 10% can increase the lifespan by up to five times.
Lithium-ion batteries, including LiFePO4, are less sensitive to deep discharges. However, maintaining a DoD below 80% is still recommended to optimize battery health. By managing DoD effectively, you can enhance battery performance and reduce replacement costs.
3.2 Influence of Operating Temperature and Environmental Conditions
Temperature plays a critical role in determining battery cycle life. High temperatures accelerate chemical reactions, leading to faster degradation. For example, the following table about effect of temperature on the aging rate of Li-ion battery operating:
Temperature (°F) | Performance Decrease (%) over 200 cycles |
---|---|
77 | 3.3 |
113 | 6.7 |
For lead-acid batteries, every 15°F increase above 77°F halves the life cycle. Operating batteries within their optimal temperature range ensures consistent battery capacity and performance. Avoid exposing batteries to extreme heat or cold to maximize their longevity.
3.3 Battery Chemistry: LCO, NCM, LiFePO4, LMO and More
Different battery chemistries exhibit varying cycle life characteristics. Here’s a comparison:
Battery Chemistry | Cycle Life (Cycles) | Notes |
---|---|---|
LiFePO4 | 2,000 – 5,000 | High safety, long life cycles |
NCM | 1,000 – 2,000 | Requires cobalt and nickel |
LCO | 500 – 1,000 | Short lifespan, low thermal stability |
LMO | 300 – 700 | Higher thermal stability than LCO |
LTO | Up to 10,000 | Very high life cycle, less polluting |
LiFePO4 batteries stand out for their exceptional cycle life and safety, making them ideal for industrial and energy storage applications. Selecting the right chemistry based on your operational needs ensures optimal battery performance.
3.4 Charging and Discharging Practices
Charging and discharging rates directly affect battery cycle life. Rapid charging or discharging generates heat, which accelerates wear and reduces battery health. Studies show that dynamic cycling, which mimics real-world usage, can extend battery life by up to 38% compared to constant current cycling. To maximize battery cycle life:
- Use chargers designed for your battery type.
- Avoid overcharging or deep discharging.
- Implement dynamic cycling profiles for better longevity.
By adopting proper charging and discharging practices, you can enhance battery capacity and ensure reliable performance over time.
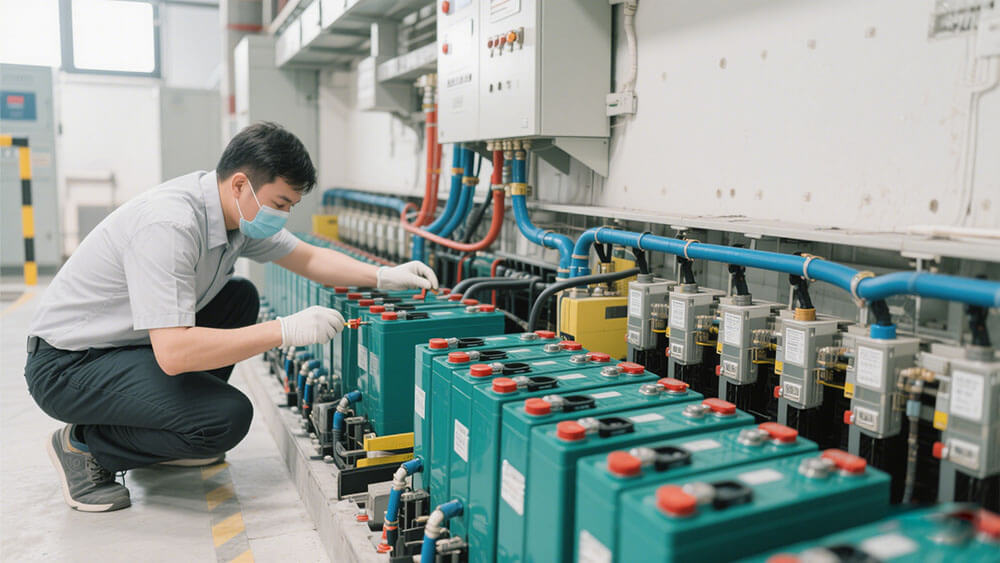
Part 4: How to Extend Battery Cycle Life
4.1 Best Practices for Charging and Discharging
Proper charging and discharging practices significantly impact battery longevity. Following these best practices can help you increase battery life:
- Store batteries at approximately 50% charge to minimize chemical degradation.
- Avoid overcharging by unplugging chargers once the battery reaches full capacity.
- Use high-quality chargers designed specifically for your battery type to prevent overheating and ensure safety.
- Maintain a Depth of Discharge (DoD) between 50% and 100%. Shallow cycles reduce stress on battery cells, extending their lifespan.
⚡ Tip: Research shows that keeping batteries within a 70% DoD range can maximize their cycle life, ensuring reliable performance over time.
4.2 Avoiding Extreme Temperatures and Environmental Stress
Temperature management is critical for preserving battery health. Extreme heat accelerates chemical reactions, leading to faster degradation, while cold temperatures temporarily reduce capacity. To protect your batteries:
- Operate them within the recommended temperature range, typically 59°F to 77°F.
- Avoid exposing batteries to direct sunlight or freezing conditions.
- Use thermal management systems for industrial battery packs to maintain optimal operating conditions.
📊 Did You Know? Lithium battery performance decreases by 3.3% at 77°F and 6.7% at 113°F over 200 cycles, highlighting the importance of temperature control.
4.3 Regular Maintenance and Monitoring of Battery Packs
Routine maintenance and monitoring ensure consistent performance and extend battery cycle life. Key practices include:
- Regularly checking the state of charge (SoC) and depth of discharge (DoD).
- Monitoring temperature and voltage levels to identify potential issues early.
- Using battery management systems (BMS) to automate monitoring and optimize performance.
Proactive maintenance reduces the risk of unexpected failures and maximizes the return on your battery investment. Having defined battery cycle life, we now explore its profound implications for real-world applications.
4.4 Selecting the Right Battery Chemistry for Specific Applications
Choosing the appropriate battery chemistry is essential for maximizing cycle life and meeting application-specific requirements. Here’s a comparison of common chemistries:
Battery Chemistry | Cycle Life (Charge Cycles) | Applications |
---|---|---|
LiFePO4 | 2,000-5,000 | Solar energy, robotics, security cameras |
NCM | 1,000-2,000 | Industrial equipment |
LCO | 500 – 1,000 | Consumer electronics |
LMO | 300-700 | Medical equipment, electric tool |
LTO | 10,000-20,000 | Network frequency modulation |
Lead-acid | 200-300 | Automotive applications |
LiFePO4 batteries stand out for their long cycle life and safety, making them ideal for energy storage and industrial use. Selecting the right chemistry ensures optimal performance and cost efficiency.
Understanding and managing battery cycle life is essential for optimizing industrial and commercial applications. The benefits of longer battery cycle life include reduced replacement costs, enhanced performance, and a smaller environmental footprint. By adopting best practices like proper charging and maintenance, you can maximize the value of your battery investments and improve operational efficiency.
FAQ
1. What is the typical cycle life of LiFePO4 batteries compared to other chemistries?
LiFePO4 batteries typically achieve 2,000-5,000 cycles, outperforming LCO (500-1,000 cycles) and NCM (1,000-2,000 cycles). Their longevity makes them ideal for industrial applications.
2. How does Depth of Discharge (DoD) affect battery cycle life?
Lower DoD levels extend cycle life. For example, reducing DoD from 80% to 50% can significantly increase the lifespan of most battery chemistries.
3. Why is temperature management crucial for battery longevity?
High temperatures accelerate chemical degradation, reducing cycle life. Operating batteries within their optimal temperature range ensures consistent performance and longer lifespan. Find a professional and reliable battery manufacturer to tailor the long longevity battery that best suits your device.