Contents
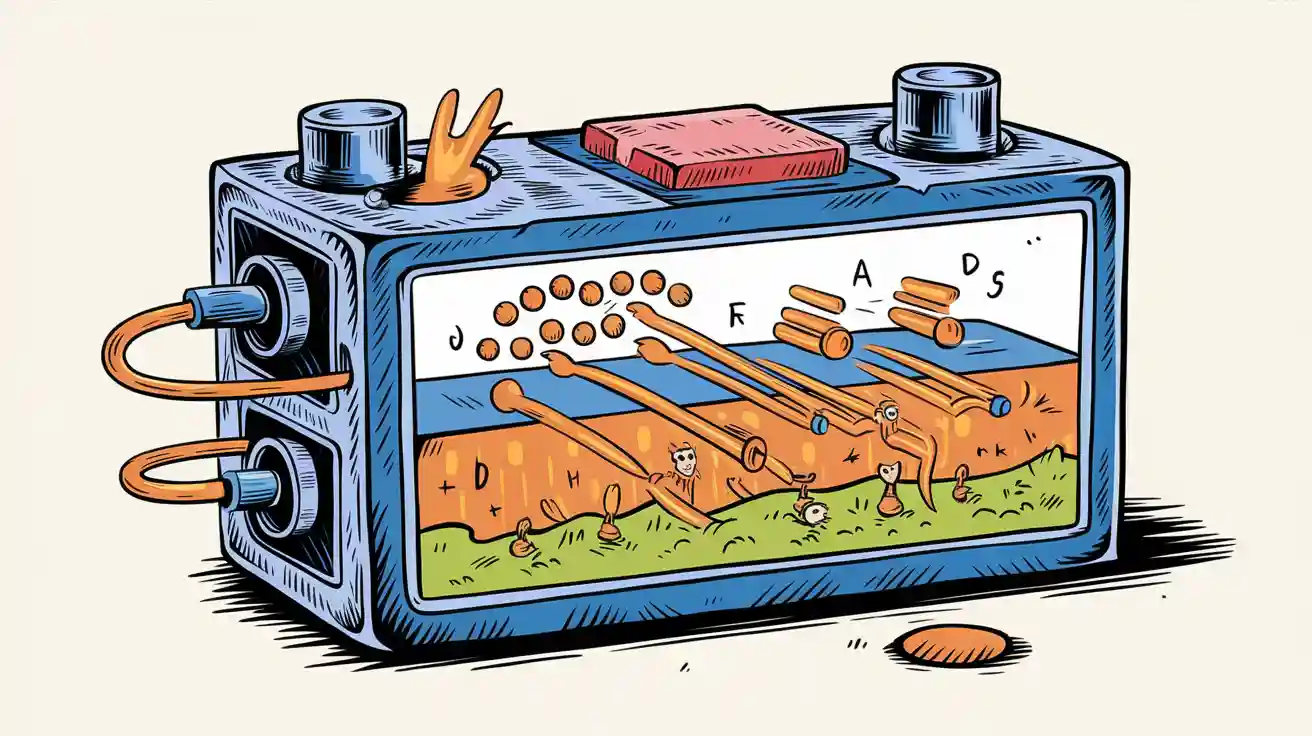
Lithium-ion batteries power modern technologies by combining advanced components to ensure efficient energy storage and delivery. Inside a lithium battery, the cathode and anode store energy, while the electrolyte facilitates ion movement. Robotics applications, projected to grow from $1.5 billion in 2023 to $4.3 billion by 2032, rely on these batteries for their high energy density and long cycle life. This makes them indispensable for industries requiring durable and reliable power solutions.
Key Takeaways
Knowing what the cathode and anode do is important. They affect how much energy the battery holds and its power.
Improving the electrolyte helps ions move better, making batteries work well. This is key for devices needing steady energy storage.
Using good materials for battery parts makes them last longer. This is important for industries wanting long-lasting batteries.
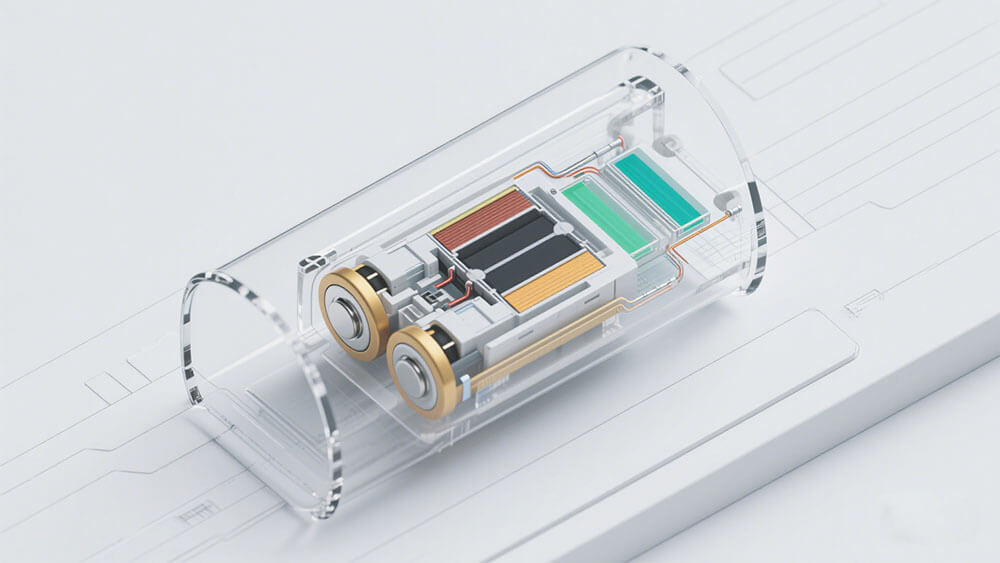
Part 1: Core Components Inside a Lithium Battery
1.1 Cathode: The Positive Electrode
The cathode serves as the positive electrode in a lithium-ion battery, playing a pivotal role in determining the battery’s energy density and voltage. It is typically composed of lithium-based compounds such as NMC (Nickel Manganese Cobalt) or LCO (Lithium Cobalt Oxide). These materials store lithium ions during the charging process and release them during discharge, enabling energy transfer.
Recent studies highlight the importance of cathode materials in battery performance. For instance, research on NMC cathodes reveals a direct relationship between lithium thickness and electrolyte depletion. This insight underscores the need for precise material engineering to enhance battery longevity and efficiency. By optimizing cathode materials, you can achieve higher energy densities and longer cycle lives, which are critical for applications like robotics and medical devices.
1.2 Anode: The Negative Electrode
The anode, or the negative electrode, complements the cathode by storing lithium ions during discharge and releasing them during charging. Graphite is the most commonly used anode material due to its stability and affordability. However, advancements in anode materials, such as silicon-based anodes, offer significantly higher capacities. Silicon anodes can store more lithium ions, but they face challenges like volume expansion, which can impact performance.
Component | Findings | Implications |
---|---|---|
Anodes | Silicon-based anodes can provide greater capacities than graphite. | Improved charge capacity but may lead to performance losses due to volume changes. |
For industrial applications, selecting the right anode material is crucial. It ensures optimal energy storage and discharge rates, which directly affect the battery’s overall performance.
1.3 Electrolyte: The Ion Conductor
The electrolyte facilitates the movement of lithium ions between the cathode and anode, acting as the medium for ion conduction. Commonly used electrolytes include liquid solutions containing lithium salts like LiPF6, dissolved in organic solvents such as ethylene carbonate (EC) and propylene carbonate (PC). The formulation of the electrolyte significantly impacts the battery’s ionic conductivity and overall efficiency.
Research has shown that varying the mass ratios of EC to PC while maintaining fixed ratios for other components can influence conductivity. For example, increasing the concentration of conducting salts from 0.2 to 2.1 mol/kg enhances ion transfer rates, improving battery performance. This makes electrolyte optimization a key factor in developing high-performance lithium-ion cells.
1.4 Separator: The Safety Barrier
The separator is a thin, porous membrane placed between the cathode and anode to prevent direct contact while allowing lithium ions to pass through. This component is critical for ensuring the safety and reliability of lithium-ion batteries. High-quality separators reduce the risk of short circuits and thermal runaway, which are essential for applications in sensitive environments like medical devices.
Modern separators are designed with advanced materials to enhance thermal stability and mechanical strength. By investing in robust separator technology, you can improve the safety and lifespan of your battery systems.
1.5 Current Collectors: Ensuring Efficient Energy Flow
Current collectors, typically made of aluminum for the cathode and copper for the anode, facilitate the flow of electrons between the electrodes and the external circuit. These components play a vital role in minimizing energy losses and ensuring efficient power delivery.
Aluminum current collectors are prone to corrosion over time, which can degrade battery performance.
Corrosion affects the solid/solid interface, leading to a 1.14% loss in Coulomb efficiency.
Stress corrosion cracks may develop at higher charging voltages, impacting the passivation layer and causing capacity damage exceeding 20%.
To mitigate these issues, you should consider advanced designs and coatings for current collectors. This ensures durability and consistent performance, especially in demanding industrial and infrastructure applications.
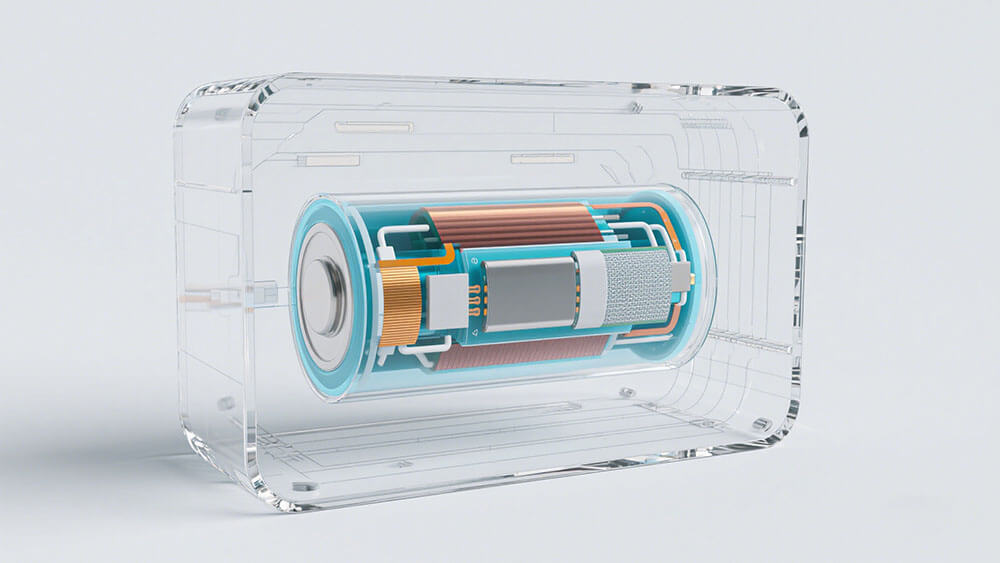
Part 2: How Lithium-Ion Battery Components Work Together
2.1 The Charging Process: Lithium-Ion Movement and Energy Storage
The charging process in a lithium-ion battery involves the movement of lithium ions from the cathode to the anode through the electrolyte. This process stores energy by embedding lithium ions into the anode material, typically graphite. The efficiency of this energy storage depends on the interaction between battery components, including the electrolyte, separator, and electrodes.
Scientific studies have quantified key parameters influencing energy transfer efficiency during charging. These include characteristic time, diffusion coefficients, and electrolyte conductivities. The table below summarizes these parameters:
Parameter | Description |
---|---|
Characteristic time | Associated with charge/discharge, linked to electrode/electrolyte properties via rate-limiting processes. |
Diffusion coefficients | Derived from fitting equations to capacity versus rate data, indicating ionic movement efficiency. |
Electrolyte conductivities | Parameters that influence energy transfer efficiency during the charging process. |
Advanced techniques like NMR spectroscopy provide insights into lithium-ion dynamics. These methods reveal the formation of the solid-electrolyte interphase (SEI) and monitor dendritic growth, which are critical for understanding battery performance. By optimizing the electrolyte and electrode materials, you can enhance the efficiency of lithium-ion movement, ensuring reliable energy storage for applications like industrial systems and medical devices.
2.2 The Discharging Process: Powering Industrial and Consumer Applications
During discharge, lithium ions move back from the anode to the cathode, releasing stored energy to power devices. This process is crucial for applications ranging from industrial machinery to consumer electronics. The seamless interaction of battery components, including the separator and current collectors, ensures efficient energy delivery.
Performance curves and statistical data validate the operational efficiency of lithium-ion batteries in diverse scenarios. Key factors include the state of health (SOH), capacity, and internal resistance. The table below highlights these factors:
Factor | Description |
---|---|
State of Health (SOH) | Compares the current state of the battery to a new battery at its beginning of life (BOL). |
Capacity | Aging-induced reduction leads to decreased range of Battery Electric Vehicles (BEVs). |
Internal Resistance | Changes with aging, affecting overall battery performance. |
Impact of Aging | Affects capacity and internal resistance, crucial for applications like grid energy storage. |
Empirical evidence shows that lithium-ion cells maintain stable estimation performance even as they age. For instance:
The best root-mean-square error (RMSE) achieved is 0.011 on cell #3 with n = 0/1.
The average RMSE on the test set is 0.0156, with minimal fluctuations across datasets.
These findings demonstrate the reliability of lithium-ion batteries in powering critical systems, including robotics and infrastructure applications.
2.3 Material Selection and Its Impact on Battery Performance
The choice of materials for battery components significantly impacts performance, lifespan, and recyclability. Cathode materials like NMC and LCO determine energy density and voltage, while anode materials such as graphite and silicon influence capacity and cycle life. For example, NMC Lithium batteries offer a platform voltage of 3.5–3.6V and an energy density of 160–270Wh/kg, making them ideal for high-performance applications.
Empirical studies highlight the importance of material selection in optimizing battery performance. The table below summarizes key findings:
Methodology | Findings | Implications |
---|---|---|
Structural Equation Modeling (SEM) and Analytic Hierarchy Process (AHP) | Investigated the impact of LIB design on recycling efficiency | Highlights the importance of material selection in optimizing recycling processes |
Data Collection from 15 industry experts and 150 facilities | Analyzed relationships between design complexity and recycling performance | Provides insights into how material choices affect overall battery performance |
Multi-group analysis of different battery types | Demonstrated superior recyclability of CTP and CTB designs | Suggests that material selection can enhance recycling efficiency and reduce lifecycle costs |
By selecting high-quality materials for the cathode, anode, and separator, you can improve the efficiency and sustainability of lithium-ion cells. This is particularly important for industries prioritizing long-term performance and environmental responsibility. For more insights into sustainable battery solutions, visit Sustainability at Large Power.
Lithium-ion batteries depend on the seamless interaction of their components to deliver reliable energy storage and power. Each component, including the cathode, anode, electrolyte, separator, and current collectors, plays a critical role in ensuring efficiency and safety. For example, integrating a safety reinforced layer (SRL) has reduced battery explosion risks from 63% to 10%, highlighting advancements in safety. Understanding these components enables you to optimize energy storage solutions for applications like electric vehicles and industrial systems. For tailored lithium-ion battery solutions, visit Large Power’s Custom Battery Solutions.
FAQ
1. What makes lithium-ion batteries suitable for industrial applications?
Lithium-ion batteries offer high energy density, long cycle life, and reliability. These features make them ideal for powering industrial systems. Learn more about industrial applications.
2. How does the separator enhance battery safety?
The separator prevents direct contact between electrodes while allowing ion flow. This reduces short-circuit risks, ensuring safety in sensitive environments like medical devices.
3. Can Large Power provide custom lithium-ion battery solutions?
Yes, Large Power specializes in tailored lithium-ion battery solutions for diverse industries. Explore our custom battery solutions to meet your specific needs.