Contents
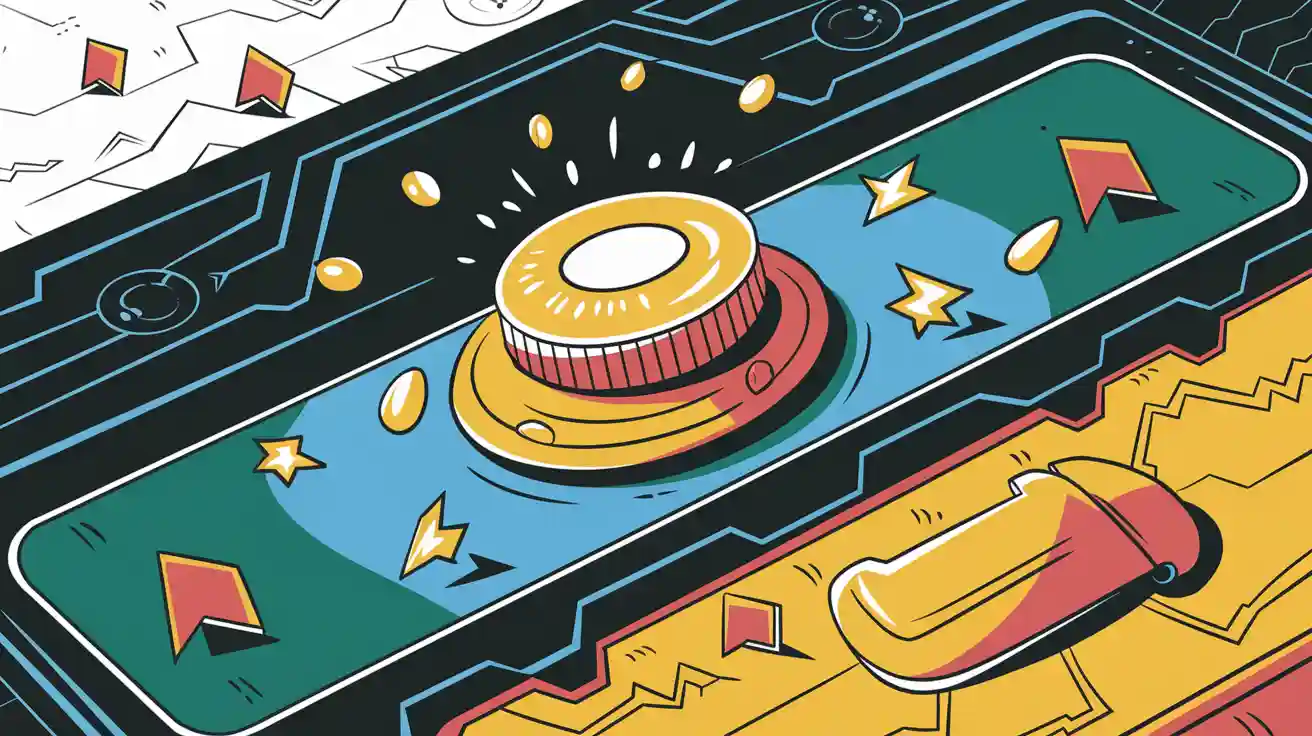
Batteries operate by storing chemical energy and converting it into electrical energy during discharge. This process relies on internal chemical reactions. When charging, energy is stored; when used, it is released. Lithium battery packs, a popular solution, dominate industrial and commercial sectors. For example:
Automotive manufacturers increasingly use lithium-ion batteries in hybrid vehicles.
Public transportation systems adopt lithium-powered buses to cut costs and emissions.
Material handling equipment, like forklifts, is transitioning to lithium-ion technology, with projections indicating widespread adoption by 2028.
This growth highlights the importance of understanding the type of energy of a battery and its applications.
Key Takeaways
Batteries keep chemical energy and change it into electrical energy. This makes them important for running devices and systems.
Lithium-ion batteries are well-liked because they store a lot of energy and work well. They are used in many things, like gadgets and factory machines.
Knowing how batteries change energy can improve how they work. It can also make them last longer and store energy better.
Part 1: Understanding the Type of Energy of a Battery
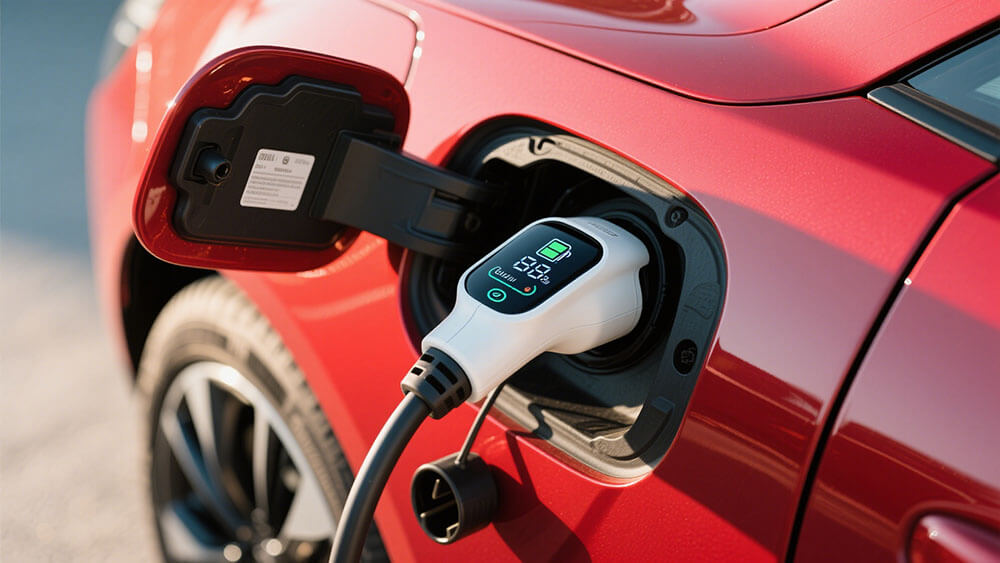
1.1 What is the type of energy stored in a battery?
Batteries store chemical energy, which is later converted into electrical energy to power devices and systems. This type of energy storage is achieved through electrochemical reactions within the battery’s cells. In lithium-ion batteries, for instance, lithium ions move between the anode and cathode during charging and discharging. This movement facilitates the storage and release of energy in a highly efficient manner.
The type of energy in a battery is unique because it combines chemical and electrical properties. Chemical energy in batteries is stored in the bonds of molecules, while electrical energy is generated when these bonds are broken during discharge. This dual nature makes batteries versatile for various applications, from powering consumer electronics to supporting industrial infrastructure.
1.2 Why chemical energy is ideal for energy storage
Chemical energy is ideal for energy storage due to its high energy density and reliability. Lithium-ion batteries, for example, offer energy densities ranging from 160 to 270 Wh/kg, making them suitable for applications requiring compact and lightweight solutions. Additionally, chemical energy can be stored for extended periods without significant loss, ensuring consistent performance over time.
Another advantage of chemical energy is its scalability. Whether you need a small battery for a medical device or a large battery pack for industrial equipment, chemical energy storage systems can be customized to meet specific requirements. This flexibility is particularly valuable in industries like robotics and transportation, where energy demands vary widely.
Tip: To optimize the performance of lithium-ion batteries, consider factors like temperature control and proper charging practices. These measures can enhance the efficiency of energy storage and prolong the battery’s lifespan.
1.3 How chemical energy is converted to electrical energy
The conversion of chemical energy to electrical energy in a battery involves a series of electrochemical reactions. During discharge, the anode releases electrons, which travel through an external circuit to the cathode. This flow of electrons generates electricity, powering connected devices. Simultaneously, lithium ions move through the electrolyte from the anode to the cathode, maintaining charge balance within the battery.
The efficiency of this conversion process depends on several factors, including the battery’s design and operating conditions. For instance, temperature fluctuations can significantly impact the conductivity of the electrolyte, affecting the overall performance. Recent studies have highlighted the importance of precise temperature management in minimizing data uncertainty and optimizing battery aging.
Evidence Description | Key Findings |
---|---|
Differential techniques for data uncertainty | Techniques like dCapacity/dncyc and dK/dncyc reveal the magnitude of uncertainty in battery aging. |
SRE regression results | R² values generally exceed 0.995, indicating high confidence in aging rate analyses. |
Impact of temperature fluctuations | Temperature has a more significant effect on conductance data uncertainty than measurement errors. |
Understanding the conversion process is crucial for industries relying on lithium-ion batteries. By optimizing this process, you can achieve higher efficiency and reliability, ensuring that your energy storage systems meet the demands of modern applications.
Part 2: The Energy Conversion Process in Battery Storage
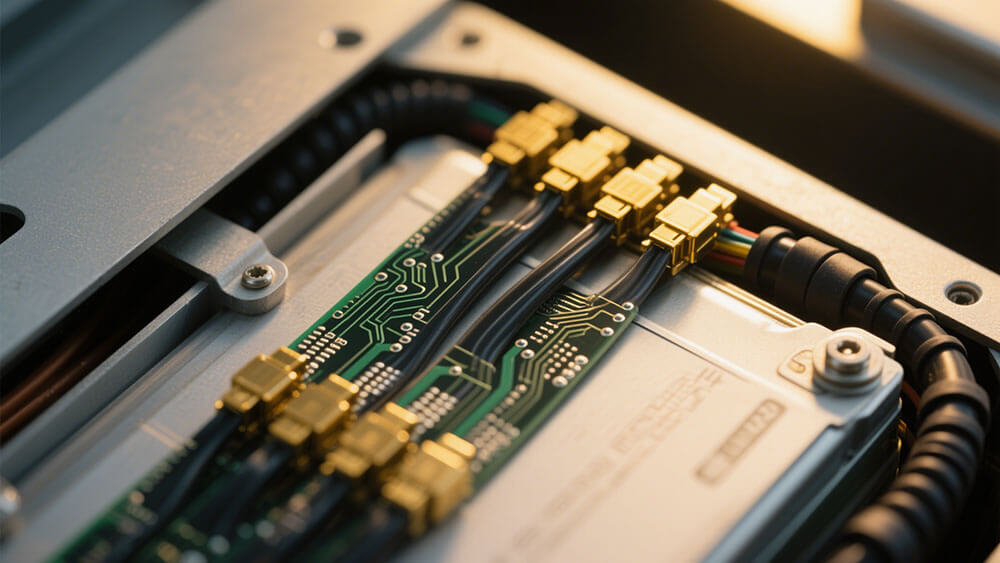
2.1 The structure of a lithium battery pack and the chemical reactions enabling energy conversion
Lithium battery packs consist of multiple cells, each containing an anode, cathode, electrolyte, and separator. The anode, typically made of graphite, stores lithium ions during charging. The cathode, composed of materials like NMC or LMO, facilitates the release of these ions during discharge. The electrolyte enables ion movement between the electrodes, while the separator prevents direct contact, ensuring safe operation.
Chemical reactions within these components drive energy conversion. Lithium insertion mechanisms at the cathode and anode impact voltage and energy density. For example, the spinel structure of LMO Lithium batteries enhances lithium-ion diffusion, improving performance.
Aspect | Details |
---|---|
Cathode Materials | Transition from simple oxide to polyanion oxide cathodes, enhancing energy density and operating voltage. |
Structural Characteristics | Spinel structure of LiMn2O4 with specific ion placements facilitating lithium-ion diffusion. |
Chemical Reactions | Lithium insertion mechanisms and their impact on voltage and energy conversion processes. |
Understanding these structural and chemical dynamics helps you optimize energy storage systems for applications like robotics and infrastructure.
Note: For custom lithium battery solutions tailored to your industry needs, explore Large Power’s offerings.
2.2 The flow of electrons and creation of electrical energy
Electrical energy generation in lithium-ion batteries relies on electron flow. During discharge, oxidation occurs at the anode, releasing electrons. These electrons travel through an external circuit to the cathode, where reduction takes place. This movement creates electricity, powering devices. Simultaneously, lithium ions move through the electrolyte, maintaining charge balance.
In galvanic cells, spontaneous chemical reactions drive this process. For lithium-ion batteries, compounds like lithium cobalt oxide or lithium manganese oxide facilitate efficient energy conversion. This principle applies across industries, from consumer electronics to industrial equipment.
Tip: Proper battery management systems (BMS) can enhance electron flow efficiency and prolong battery life. Learn more about BMS here.
2.3 Efficiency and challenges in lithium battery energy conversion
Lithium battery energy conversion achieves high efficiency, with utility-scale systems in the U.S. reporting round-trip efficiencies of 82%. However, challenges like temperature sensitivity and electrolyte degradation can impact performance.
Efficiency Metrics:
Utility-scale battery fleet (2019): 82%
Pumped-storage facilities (2019): 79%
Phase conversion pathways at the nanoscale reveal mechanisms affecting efficiency. For instance, unidirectional phase propagation in nanosheets demonstrates how chemical reactions influence energy conversion.
Evidence Description | Key Findings |
---|---|
Phase conversion pathways in lithium-ion batteries | Understanding nanoscale pathways can reveal mechanisms and challenges of conversion reactions. |
XAS in Auger electron yield mode | AEY intensity changes with surface overlayer thickness, indicating electrochemical processes during charging and discharging. |
Unidirectional phase propagation mechanism | Valid under relevant battery conditions, with multiple propagation fronts merging in nanosheets. |
To overcome these challenges, you can implement advanced cooling systems and monitor electrolyte health. These measures ensure reliable energy storage and conversion for demanding applications.
Call to Action: For tailored solutions addressing efficiency challenges, consult Large Power’s experts.
Part 3: How Batteries Store and Release Energy
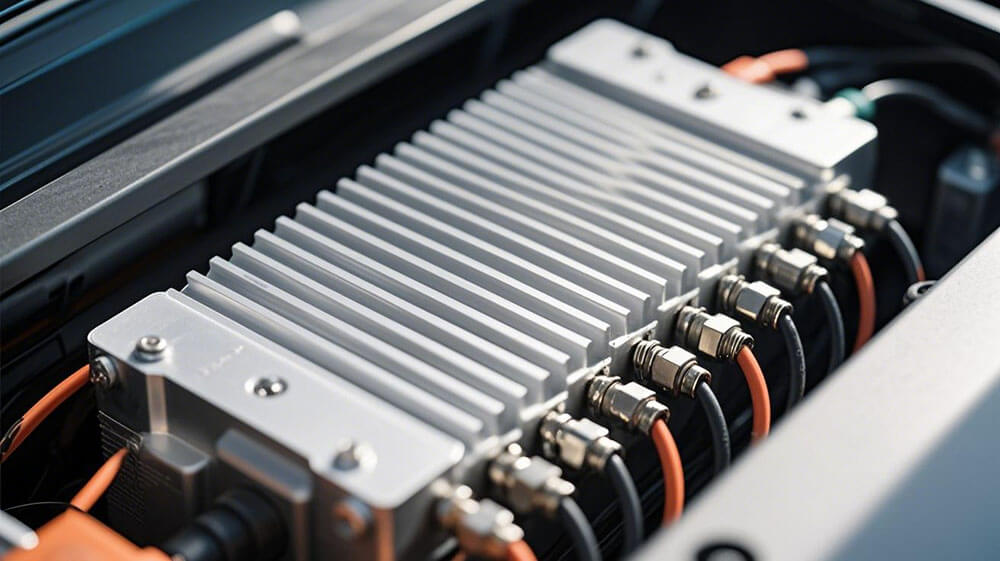
3.1 The chemical reactions enabling energy conversion
Batteries rely on chemical reactions to store and release energy effectively. In lithium-ion batteries, these reactions occur between the anode, cathode, and electrolyte. During charging, lithium ions move from the cathode to the anode through the electrolyte, where they are stored. When the battery discharges, the ions flow back to the cathode, releasing stored energy in the form of electricity.
The efficiency of these reactions depends on the materials used in the battery. For example, NMC Lithium batteries utilize nickel, manganese, and cobalt in their cathodes, offering high energy density and long cycle life. Similarly, LMO Lithium batteries use a spinel structure that enhances lithium-ion diffusion, improving performance.
Recent advancements in battery technology have introduced three-dimensional electrode architectures. These designs increase the surface area for chemical reactions, enhancing energy storage and release capabilities. Researchers are also employing diagnostic tools like X-ray spectroscopy to monitor these reactions in real-time, providing valuable insights into battery performance.
Understanding these chemical processes is crucial for optimizing battery storage systems, especially in industries like robotics and infrastructure, where reliability and efficiency are paramount.
Note: For custom lithium battery solutions tailored to your industry, consult Large Power’s experts.
3.2 The process of energy storage in lithium batteries
Energy storage in lithium batteries involves a series of precise manufacturing and operational steps. The process begins with the coating of active materials onto electrodes, followed by cell assembly and electrolyte filling. These steps ensure that the battery can store energy efficiently.
A case study conducted in Houston, Texas, explored the feasibility of a mid to large-scale Battery Energy Storage System (BESS) plant. The study highlighted the importance of quality checks during manufacturing, which are critical for ensuring the reliability of energy storage systems.
Key Manufacturing Steps:
Coating active materials onto electrodes.
Assembling cells with separators and electrolytes.
Filling cells with electrolytes and sealing them.
Conducting rigorous quality checks to ensure performance.
Once operational, lithium batteries store energy by moving lithium ions from the cathode to the anode during charging. This process creates a potential difference, which is later utilized to generate electricity during discharge. Advanced diagnostic tools now allow real-time monitoring of this process, enabling adjustments to optimize performance and extend battery life.
Tip: Implementing a robust Battery Management System (BMS) can further enhance the efficiency of energy storage. Learn more about BMS at Large Power.
3.3 The role of chemical energy in lithium battery packs
Chemical energy plays a pivotal role in the functionality of lithium battery packs. It serves as the primary medium for storing and releasing energy, making it indispensable for applications ranging from consumer electronics to industrial equipment.
In lithium-ion batteries, chemical energy is stored in the bonds of lithium compounds. When the battery discharges, these bonds break, releasing energy in the form of electricity. This process is highly efficient, with modern lithium batteries achieving energy densities of up to 270 Wh/kg.
Researchers are continuously exploring ways to improve the role of chemical energy in batteries. For instance, mathematical models are being developed to predict battery performance and optimize charge/discharge cycles. These models help in identifying potential failures, ensuring that the battery operates at peak efficiency.
Additionally, the use of X-ray absorption near edge structure (XANES) spectroscopy has revolutionized the understanding of chemical energy storage. This technique provides detailed insights into the internal state of batteries without disassembly, accelerating innovation in battery technology.
Call to Action: For tailored lithium battery solutions that maximize the potential of chemical energy, explore Large Power’s offerings.
Part 4: Measuring Energy in Lithium Battery Packs
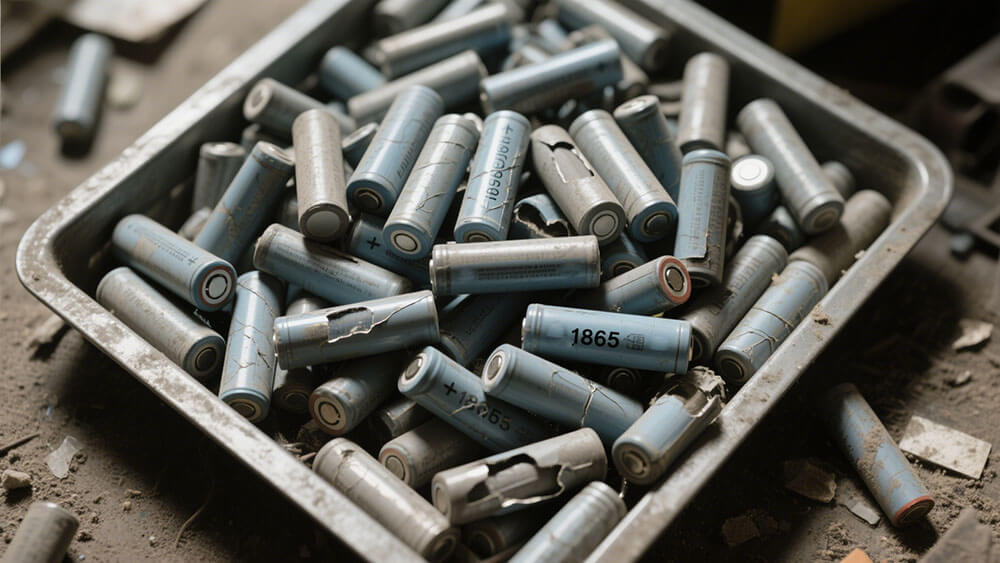
4.1 Key metrics for battery energy measurement
Measuring the performance of a lithium battery pack involves tracking several key metrics. These metrics provide insights into efficiency, reliability, and sustainability. For instance, energy density measures how much energy a battery can store relative to its weight. Higher energy density translates to lighter batteries, which is crucial for applications like robotics and transportation. Another critical metric is battery lifespan, which determines how many charge-discharge cycles a battery can endure before its capacity significantly degrades.
Cost reduction per kilowatt-hour is another essential factor. It reflects improvements in manufacturing processes and economies of scale. Monitoring production efficiency and yield ensures that most batteries produced meet quality standards, reducing waste and enhancing profitability. Environmental impact reduction is equally important, as it tracks the use of eco-friendly materials and compliance with sustainability regulations.
Metric | Benchmark Value | Real-life Application |
---|---|---|
Energy Density Improvement Rate | Enhances capacity | Drives cost reduction and market competitiveness |
Battery Lifespan | Improves reliability | Boosts customer retention and revenue stability |
Cost Reduction Per Kilowatt-Hour | 5-10% YoY | Indicates process improvements and economies of scale |
Production Efficiency and Yield | 90-95% yield | Highlights enhanced manufacturing efficiency |
Environmental Impact Reduction | 15-20% improvement | Emphasizes eco-friendly materials and regulatory compliance |
4.2 Tools and methods for assessing battery storage capacity
Accurate assessment of battery storage capacity is vital for ensuring optimal performance. Tools like electrochemical impedance spectroscopy (EIS) and incremental capacity-differential voltage analysis are widely used. These techniques help diagnose battery degradation and predict performance over time. For example, EIS measures the internal resistance of a battery, providing insights into its health.
Temperature regulation also plays a significant role. Studies show that a 15°C temperature difference can cause a 5% capacity loss, highlighting the importance of maintaining stable conditions. Standardized testing methods, such as frequency regulation duty-cycles, ensure consistent and reliable evaluations of energy storage systems.
Effective Methods:
Electrochemical impedance spectroscopy for internal resistance analysis.
Incremental capacity-differential voltage for degradation assessment.
Frequency regulation duty-cycles for standardized performance testing.
Study | Findings |
---|---|
Kim et al. (2022) | Li-Iron Phosphate batteries maintain good aging stability under cycling. |
Ganesan et al. (2016) | A 15°C temperature difference causes a 5% system capacity loss. |
4.3 Importance of energy measurement in industrial applications
Energy measurement is critical for ensuring safety and performance in industrial applications. Monitoring parameters like voltage, current, and temperature helps prevent failures such as overcharging or thermal runaway. For example, integrating sensors into lithium-ion batteries allows real-time tracking of these parameters, enhancing reliability.
In the automotive sector, rigorous testing ensures that batteries meet the demands of electric vehicles. Similarly, industries like robotics and infrastructure rely on precise energy measurements to optimize operations and reduce downtime. Safety testing, such as abuse and thermal runaway tests, ensures compliance with industry standards and protects end-users.
Tip: For custom lithium battery solutions tailored to your industrial needs, consult Large Power’s experts.
Batteries store chemical energy and convert it into electrical energy to deliver reliable power. Lithium battery packs provide efficient electrical energy output for modern applications. Understanding how batteries function helps you optimize battery backup systems for industrial and commercial use. This knowledge ensures better performance and long-term reliability.
FAQ
1. What is a solar battery, and how does it work?
A solar battery stores energy generated by a solar power system. It uses electrochemical processes to convert and store solar energy for later use.
2. How can solar energy storage improve efficiency in a solar power system?
Solar energy storage ensures consistent energy availability. It reduces reliance on the grid and optimizes the performance of your solar power system.
3. Are solar batteries suitable for industrial applications?
Solar batteries are ideal for industrial use. They provide scalable energy storage solutions, ensuring reliability and efficiency for large-scale solar power systems.
Tip: For custom lithium battery solutions tailored to your industrial needs, consult Large Power’s experts.