Contents
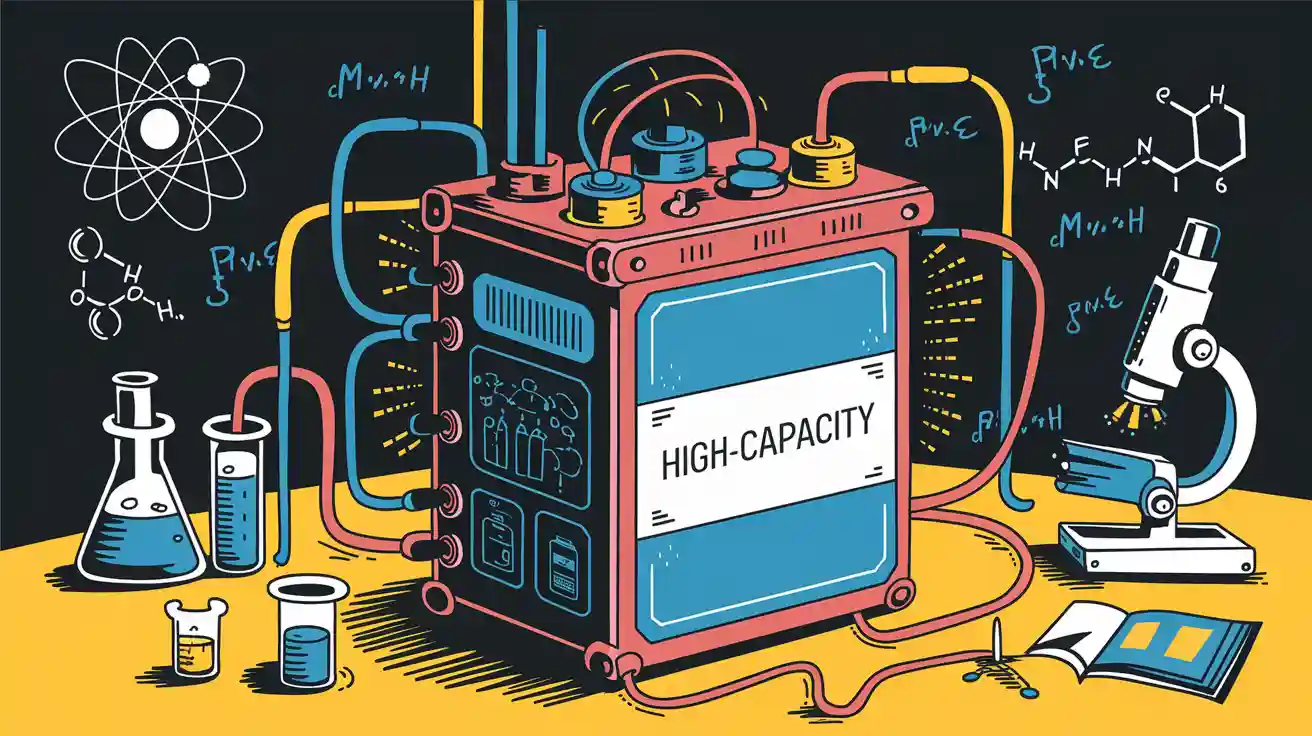
High Capacity Battery solutions, engineered to store substantial amounts of energy, are driving innovation across modern industries. Energy density, a vital metric, quantifies the energy stored per unit weight or volume, and lithium-ion batteries, with gravimetric energy density reaching 711.3 Wh/kg, are at the forefront of advancements in high energy density technologies. These High Capacity Batteries are integral to powering electric vehicles, renewable energy systems, and industrial applications, delivering unmatched efficiency and reliability. The global lithium-ion battery market for consumer electronics is projected to reach $100 billion by 2030, underscoring their critical role in energy storage solutions.
Explore custom battery solutions for tailored energy needs.
Key Takeaways
High-capacity batteries, like lithium-ion, power electric cars and renewable energy. They store a lot of energy and work efficiently.
New materials, like solid-state batteries and better electrode designs, improve how batteries work and make them safer. These changes lead to new ideas for the future.
Learning about battery parts, like anodes and cathodes, helps pick the best batteries for different industries.
Part 1: The Basics of High-Capacity Battery Performance
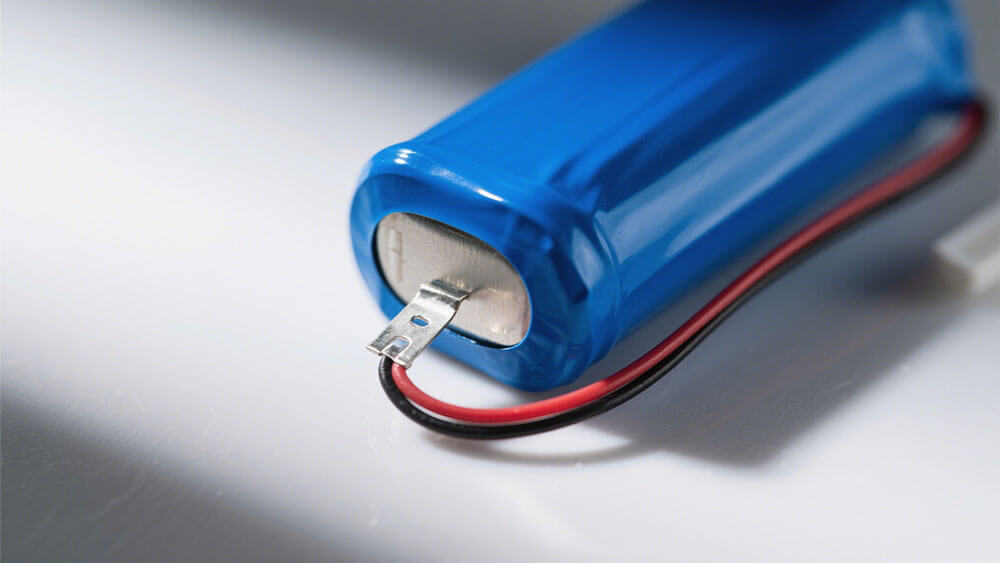
1.1 Key Components: Anode, Cathode, and Electrolyte
High-capacity batteries rely on three critical components: the anode, cathode, and electrolyte. Each plays a distinct role in ensuring optimal energy density and performance. The anode, typically made of graphite or other carbon-based materials, serves as the host for lithium ions during the charging process. It stabilizes the solid-electrolyte-interphase (SEI), a protective layer that enhances the battery’s efficiency and longevity. The cathode, often composed of high-capacity materials like LiNi0.5Mn1.5O4, facilitates the movement of lithium ions back to the anode during discharge. However, this material requires specific electrolyte formulations to maintain stability and prevent degradation.
The electrolyte acts as the medium through which lithium ions travel between the anode and cathode. Reformulated electrolytes are now designed to stabilize both interfaces, improving the overall performance of lithium-ion batteries. These advancements in material science have significantly contributed to the development of better batteries with higher energy density and longer lifespans.
Key Component | Function Description |
---|---|
Anode | Stabilizes the solid-electrolyte-interphase, enhancing performance in lithium-ion batteries. |
Cathode | Utilizes LiNi0.5Mn1.5O4, a high-capacity material, requiring specific electrolyte formulations for stability. |
Electrolyte | Reformulated to stabilize both anode and cathode interfaces, improving overall battery performance. |
1.2 How Energy is Stored and Released in Lithium-Ion Batteries
The energy storage and release process in lithium-ion batteries is a sophisticated yet efficient mechanism. During charging, lithium ions move from the cathode to the anode through the electrolyte. These ions intercalate into the anode’s graphite structure without causing distortion, ensuring the battery’s structural integrity. When the battery discharges, the process reverses. Lithium atoms in the anode ionize, releasing electrons that travel through an external circuit to power devices. Simultaneously, lithium ions migrate back to the cathode, where they recombine with electrons, neutralizing their charge.
This seamless movement of ions and electrons is the foundation of lithium-ion battery technology. The process generates free electrons in the anode, creating an electric current that powers applications ranging from consumer electronics to industrial systems. The ability to store and release energy efficiently makes lithium-ion batteries a cornerstone of modern energy solutions.
Key steps in the energy storage and release process:
Lithium atoms in the anode ionize and move through the electrolyte to the cathode during discharge.
Lithium ions recombine with electrons at the cathode, neutralizing their charge.
The intercalation process allows lithium ions to be stored within graphite anodes without structural distortion.
The movement of lithium ions generates free electrons in the anode, creating an electric current.
These processes highlight the importance of optimizing battery components to achieve higher weight-based energy density and volume-based energy density. By refining the chemistry and design of lithium-ion batteries, manufacturers can deliver high-capacity battery solutions tailored to diverse industrial needs.
Explore custom battery solutions for tailored energy needs.
Part 2: Factors Influencing Energy Density in Batteries
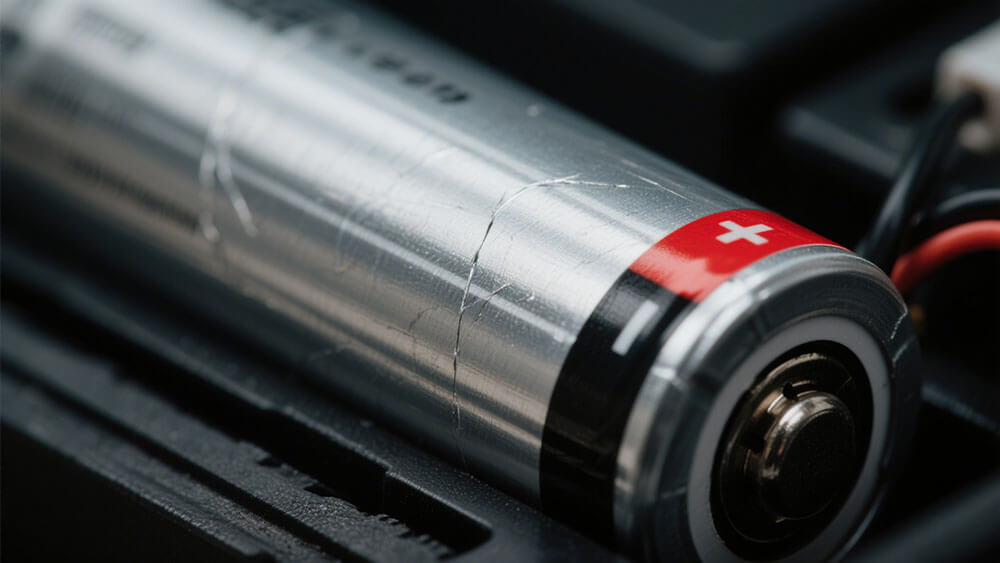
2.1 Material Science Advancements: Lithium-Ion and Solid-State Batteries
Material science plays a pivotal role in enhancing the energy density of batteries. Lithium-ion batteries, widely recognized for their high capacity and efficiency, have undergone significant advancements in recent years. By optimizing the chemical composition of the cathode and anode materials, researchers have achieved higher specific energy and improved cycle life. For instance, NMC Lithium batteries, with a platform voltage of 3.6–3.7V and energy density ranging from 160–270 Wh/kg, offer a balance between performance and longevity. Similarly, LiFePO4 Lithium batteries, known for their stability and safety, provide energy densities of 100–180 Wh/kg and cycle capabilities of up to 5000 cycles.
Solid-State Batteries represent another breakthrough in material science. These batteries replace liquid electrolytes with solid ones, enhancing safety and enabling higher energy densities of 300–500 Wh/kg. The absence of flammable liquid electrolytes reduces the risk of thermal runaway, making them ideal for applications requiring high reliability, such as medical devices and robotics. Solid-State Batteries also support faster charging and longer lifespans, addressing key limitations of traditional lithium-ion technology.
Battery Type | Platform Voltage | Energy Density (Wh/kg) | Cycle Life (Cycles) |
---|---|---|---|
NMC Lithium battery | 3.6–3.7V | 160–270 | 1000–2000 |
LiFePO4 Lithium battery | 3.2V | 100–180 | 2000–5000 |
Solid-State Battery | N/A | 300–500 | N/A |
These advancements in material science not only improve energy density but also contribute to sustainability by reducing the environmental impact of battery production. For more insights into sustainable practices in battery manufacturing, visit Sustainability at Large Power.
2.2 Electrode Design and Chemical Composition
Electrode design and chemical composition are critical factors influencing the energy density of batteries. The anode and cathode materials must be engineered to maximize lithium-ion storage while minimizing structural degradation. For example, graphite anodes offer excellent weight-based energy density due to their ability to intercalate lithium ions without distortion. Cathodes, on the other hand, benefit from high-capacity materials like LiNi0.5Mn1.5O4, which enhance volume-based energy density.
Innovative electrode designs, such as nanostructured materials, further improve battery performance. Nanotechnology enables the creation of electrodes with larger surface areas, facilitating faster ion transfer and reducing internal resistance. This results in better batteries with higher energy density and improved efficiency. Additionally, advanced chemical compositions, such as silicon-based anodes, promise even greater specific energy by accommodating more lithium ions per unit weight.
By leveraging these advancements, you can develop high-capacity battery solutions tailored to industrial applications, including infrastructure and security systems. For customized solutions, explore Custom Battery Solutions.
Part 3: Challenges and Innovations in High-Capacity Batteries
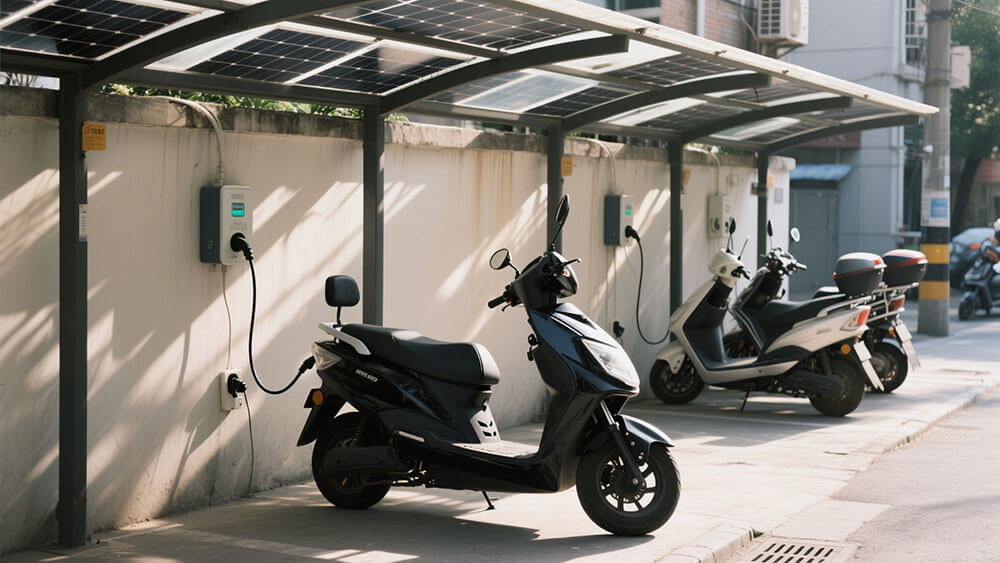
3.1 Limitations of Current Lithium-Ion Technologies
Despite their widespread adoption, lithium-ion batteries face several challenges that limit their performance and scalability in high-demand applications like electric vehicles and renewable energy storage. These limitations stem from material properties, design constraints, and chemical inefficiencies.
Limitation Type | Cause/Influence |
---|---|
Cycling Rate | Faster cycling reduces available energy, impacting long-term reliability. |
Active Material Particle Size | Larger particles decrease energy availability and slow ion transfer. |
Species Diffusivity | Low diffusivity restricts performance during high-rate discharges. |
Electrode Thickness | Thicker electrodes exacerbate diffusion limitations, reducing energy density. |
Initial Salt Concentration | Lower concentrations (e.g., 1 mol•L−1) worsen diffusion issues, especially in high-capacity systems. |
Charge Transport | Solid-phase diffusion and charge transport inefficiencies hinder overall battery performance. |
These factors collectively reduce the weight-based energy density and volume-based energy density of lithium-ion batteries, making it challenging to meet the growing energy storage demands of electric vehicles and industrial systems. Addressing these issues requires innovative approaches to battery design and material science.
3.2 Emerging Solutions: Nanotechnology and Alternative Materials
Nanotechnology and alternative materials are revolutionizing the development of better batteries with higher energy density and improved durability. Researchers are exploring silicon anodes, which offer a theoretical capacity over ten times greater than traditional graphite anodes. This advancement could significantly extend the lifespan of batteries used in electric vehicles and renewable energy systems. However, silicon anodes tend to swell and crack during charge-discharge cycles. To mitigate this, scientists have developed nanoscale designs, such as hollow nanotubes and graphene cages, which allow silicon particles to expand and contract without damaging the battery structure.
Other innovations include solid-state batteries, which replace liquid electrolytes with solid ones. These batteries enhance safety and achieve energy densities of 300–500 Wh/kg, making them ideal for applications in medical devices and robotics. Additionally, advanced cathode materials like LiNi0.5Mn1.5O4 are being optimized to improve specific energy and cycle life.
Key advancements in nanotechnology and materials:
Silicon anodes with nanoscale designs prevent structural degradation.
Solid-state batteries eliminate flammable electrolytes, enhancing safety.
High-capacity cathode materials improve energy storage efficiency.
These breakthroughs promise to overcome the limitations of current lithium-ion technologies, paving the way for high-capacity battery solutions tailored to diverse industrial needs. For customized solutions, explore Custom Battery Solutions.
Part 4: Real-World Applications and Future Trends
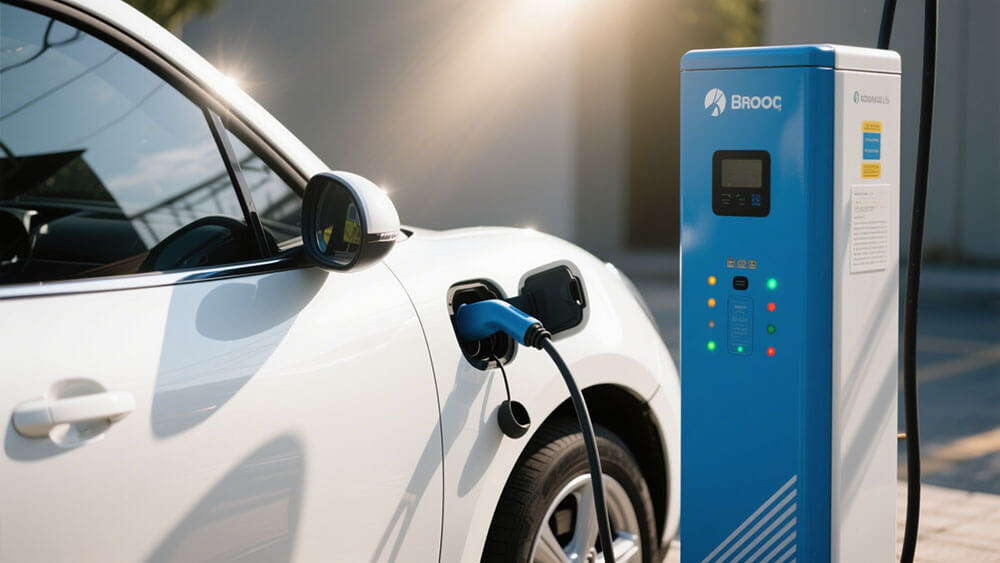
4.1 Applications in Electric Vehicles, Renewable Energy, and Industrial Systems
High-capacity batteries are transforming industries by enabling efficient energy storage and delivery. In electric vehicles (EVs), these batteries provide extended range and faster charging capabilities, addressing critical consumer demands. NMC Lithium batteries, with energy densities of 160–270 Wh/kg, are widely used in EVs due to their balance of performance and cycle life. Similarly, LiFePO4 Lithium batteries offer superior safety and durability, making them ideal for heavy-duty industrial systems.
Renewable energy systems rely on high-capacity batteries to store excess power generated during peak production. These batteries stabilize grids by supplying energy during high-demand periods, ensuring uninterrupted power delivery. Large-scale battery storage projects, such as Tesla’s Megapack installations, highlight the growing importance of batteries in achieving energy transition goals.
Industrial applications benefit from the lightweight and compact nature of high-capacity batteries. Their ability to deliver consistent power over extended periods makes them indispensable for robotics, infrastructure, and security systems. For example, robotics systems equipped with advanced lithium-ion batteries can operate autonomously for longer durations, enhancing productivity in manufacturing environments.
Aspect | Details |
---|---|
Market Growth Drivers | Rise of EV adoption and increasing demand for effective energy storage systems. |
Key Applications | Electric vehicles, energy storage systems, consumer electronics, large-scale energy applications. |
Battery Technologies | Advancements in lithium-ion batteries and other technologies. |
Market Segmentation | By type (primary/secondary), power capacity, self-discharge rate, technology, end-user, geography. |
Forecast Scenarios | Conservative, base, and optimistic scenarios for market evolution from 2024–2035. |
Major Companies | BYD and A123 Systems are notable manufacturers in the EV battery market. |
Explore tailored solutions for industrial applications at Custom Battery Solutions.
4.2 Predictions for the Future of High-Capacity Battery Technology
The future of high-capacity batteries promises significant advancements in energy density and affordability. By 2030, the price per kilowatt-hour (kWh) for automotive cells is expected to drop from $160 to $80, making EVs more accessible to a broader market. Lithium-ion battery manufacturing capacity will grow sixfold, ensuring supply meets the rising global demand projected to reach 2,722 GWh by 2030.
Solid-State Batteries will play a pivotal role in this evolution. Their energy density of 300–500 Wh/kg and enhanced safety features make them ideal for applications requiring reliability, such as medical devices and robotics. Additionally, emerging technologies like silicon-based anodes and nanostructured electrodes will further improve battery life and efficiency.
The integration of batteries into renewable energy systems will accelerate the transition to sustainable power sources. High-capacity batteries will store energy more effectively, reducing reliance on fossil fuels and supporting global decarbonization efforts. For insights into sustainable practices in battery manufacturing, visit Sustainability at Large Power.
As the market evolves, you can expect better batteries tailored to specific industrial needs. Whether you require solutions for EVs, renewable energy, or robotics, Large Power offers customized options to meet your requirements. Learn more at Custom Battery Solutions.
High-capacity batteries rely on advanced scientific principles to achieve exceptional energy density and performance. Ongoing research continues to address challenges, driving innovation in materials and designs. These advancements unlock transformative potential for industries, from renewable energy to robotics. Explore tailored battery solutions to meet your unique requirements at Custom Battery Solutions.
FAQ
1. What makes lithium-ion batteries ideal for industrial applications?
Lithium-ion batteries offer high energy density, long cycle life, and low maintenance, making them suitable for industrial systems. Learn more about industrial applications.
2. How do LiFePO4 Lithium batteries compare to NMC Lithium batteries?
LiFePO4 batteries provide superior safety and cycle life (2000–5000 cycles), while NMC batteries deliver higher energy density (160–270 Wh/kg). Both excel in specific applications.
Battery Type | Energy Density (Wh/kg) | Cycle Life (Cycles) | Safety |
---|---|---|---|
LiFePO4 Lithium battery | 100–180 | 2000–5000 | High |
NMC Lithium battery | 160–270 | 1000–2000 | Medium |
3. Why choose Large Power for custom battery solutions?
Large Power specializes in tailored lithium battery solutions for diverse industries, ensuring optimal performance and reliability. Explore custom battery solutions.